Environmental Engineering Reference
In-Depth Information
fiGure 18.2
conical flasks with silver nitrate (1 mmol l
-1
) before (left) and after (right) exposure to the culture supernatant of
Phoma
glomerata
. Reprinted with permission from Ref. [27]. © 2008, The society for Applied Microbiology.
of lactate as the electron donor to metallic platinum obtaining black color from pale yellow. The distinct approximately 5-nm-
sized platinum nanoparticles were found to be deposited in periplasmic space between the inner and outer membrane of
S. algae
[10]. yong et al. have synthesized palladium nanoparticles anaerobically by using the sulfate-reducing bacterium
Desulfovibrio
desulfuricans
NcIMB 8307 through bacterium bioreduction and biocrystallization. The ionic palladium (II) converts to palla-
dium nanoparticles on the cells surface at neutral ph in the presence of exogenous electron donor [11]. Furthermore, Mann et
al. used sediments-isolated microaerophilic bacterium
Aquaspirillum magnetotacticum
for the synthesis of magnetite (Fe
3
o
4
)
nanoparticles [12].
Desulfosporosinus
sp., a Gram-positive sulfate-reducing microbe, was used for the reduction of hexavalent
uranium U (VI) to tetravalent uranium U (IV), which precipitated Uo. These Uo crystals coated on the cell surface have a size
range of 1.5-2.5 nm [13].
18.2.1.2 Bacteria-Assisted Extracellular Nanoparticle Synthesis
Metal nanoparticle synthesis by microbes depends on the
localization of the reductive components of the cell. When the cell wall-soluble secreted enzymes or reductive enzymes are
involved in the metal ion reduction process then the metal nanoparticles are obtained by the extracellular mechanism. In
comparison with intracellular accumulation, extracellular production of nanoparticles has broader applications in electronics,
optoelectronics, bioimaging, and sensor technology. so far there have been several reports on bacteria obtained for the production
of nanoparticles such as
Rhodopseudomonas capsulata
, a prokaryotic bacterium, which was used for the synthesis of spherical
Au nanoparticles in the size range of 10-20 nm by reduction of ionic Au at room temperature under neutral condition [14].
however, variation in shapes and sizes was obtained by change in ph; at ph 4.0 triangular nanoparticles of size 50-400 nm and
spherical nanoparticles of size 10-50 nm appeared. Remarkably, extracellular synthesis of silver nanoparticles with other bacteria
such as
Aeromonas
sp. sh10 [15],
Aeromonas xylinum
[16], and
Morganella
sp. was reported [17] Prasad et al. prepared titanium
nanoparticles with spherical aggregates of 40-60 nm extracellularly using the culture filtrate of
Lactobacillus
sp. at room temper-
ature [18]. Unlike in abiotic conditions, an ancient Gram-negative cyanobacterium,
Pediastrum boryanum
UTex 485, produced
Pt (II) organics and metallic platinum nanoparticles extracellularly with different morphologies such as spherical, dendritic, and
bead-like chains in the size range of 30 nm to 0.3 µm [19]. In addition to this,
Micrococcus lactilyticus
cell-free extracts were used
for production of U(IV) by the reduction of U(VI) [20].
18.2.2
nanoparticle synthesis by fungi
In recent decades, the synthesis of silver and gold nanoparticles using fungi such as
Fusarium oxysporum
[21],
Colletotrichum
sp.
[22],
Fusarium semitectum
[23],
Aspergillus fumigatus
[24],
Coriolus versicolor
[25],
Aspergillus niger
[26] has been reported.
Birla et al. reported silver nanoparticles by
Phoma glomerata
[27]. They observed black color formation indicates reduction of
silver nanoparticles (Fig. 18.2). Fungi are more beneficial than other microorganisms in various ways; for example, fungal mycelia
mesh can resist flow pressure and agitation in bioreactors compared to plant materials and bacteria. These are fastidious to grow
and easy to handle and fabricate. Also, since the nanoparticles precipitated outside the cell are devoid of unnecessary cellular
components, they can be directly used for various applications.
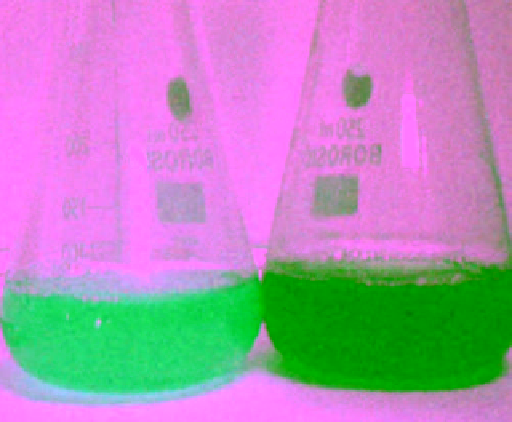