Environmental Engineering Reference
In-Depth Information
spatial incidence of the imbalance between demand and supply. We do not
entirely concur with this narrative. Water scarcity means different things to
different people in different environments. Water can be both abundant and
scarce in the same environment, depending on the kinds of water and water
uses discussed.
Some observers are blunt: “Water shortages have emerged as one of the most
important infrastructure issues in the world today . . . Global demand for
freshwater will exceed supply by 40% by 2030 . . . with potentially calamitous
implications for business, society and the environment” (KPMG, 2012).
Recent reports speak of “water bankruptcy” for many regions (Mee and
Adeel, 2012) while water shortage has been called “the defining crisis of the
21st century” (Pearce, 2007).
Scenario analysis used in the World Water Vision for 2000 warned that
continued “business as usual” water management was likely to result in, “a
global system . . . becoming more and more vulnerable as a result of the
increasing scarcity of water resources per capita, the diminished quality of water
and increasing conflicts associated with inequality, water scarcity, and the
narrower resource base of healthy ecosystems.” Scenario analysis took account
of nearly two dozen drivers of change, among them demographic, economic,
technological, social, governance, and environmental [hydrological] factors
(Gallopín and Rijsberman, 2000).
Increased demand for food (population growth and dietary changes), rapidly
growing megacities, urbanization and industrialization, biofuel production, and
the increasing effects of climate change all drive increased use of freshwater.
Demand for drinking water and sanitation services will be a factor, but the real
increase in water demand will come from agriculture to produce food, feed,
fiber and fuel. With human populations predicted to increase from 7 billion in
2012 to about 9 billion in 2050, agricultural water requirements may grow to
as much as 14,000 billion m
3
/yr (Chartres and Varma, 2011), or almost double
(see below). These predictions are based on current levels of agricultural WP,
including rainfed as well as irrigated agriculture.
These scenarios of water demand are only slightly higher than those pub-
lished in the
Comprehensive Assessment of Water in Agriculture
(CA), which noted
that, “without further improvements in water productivity or major shifts in
production patterns, the amount of water consumed by evapotranspiration
in agriculture will increase by 70%-90% by 2050. The total amount of water
evaporated in crop production would amount to 12,000-13,500 [billion
m
3
/yr], almost doubling the 7130 [billion m
3
/yr] of today” (Molden, 2007).
Other analyses estimate annual water use in agriculture at 8500-11,000
billion m
3
/yr by 2050 (Rockström et al., 2010). They assume some growth in
productivity and separate consumption in rainfed (6500-8500 billion m
3
/yr)
from irrigated (2000-2500 billion m
3
/yr) agricultural systems.
The above estimates focus on demand for agricultural water, but ignore the
supply side. How bad is the mismatch between demand and supply of
agricultural water? We discuss three ways to address this question—through
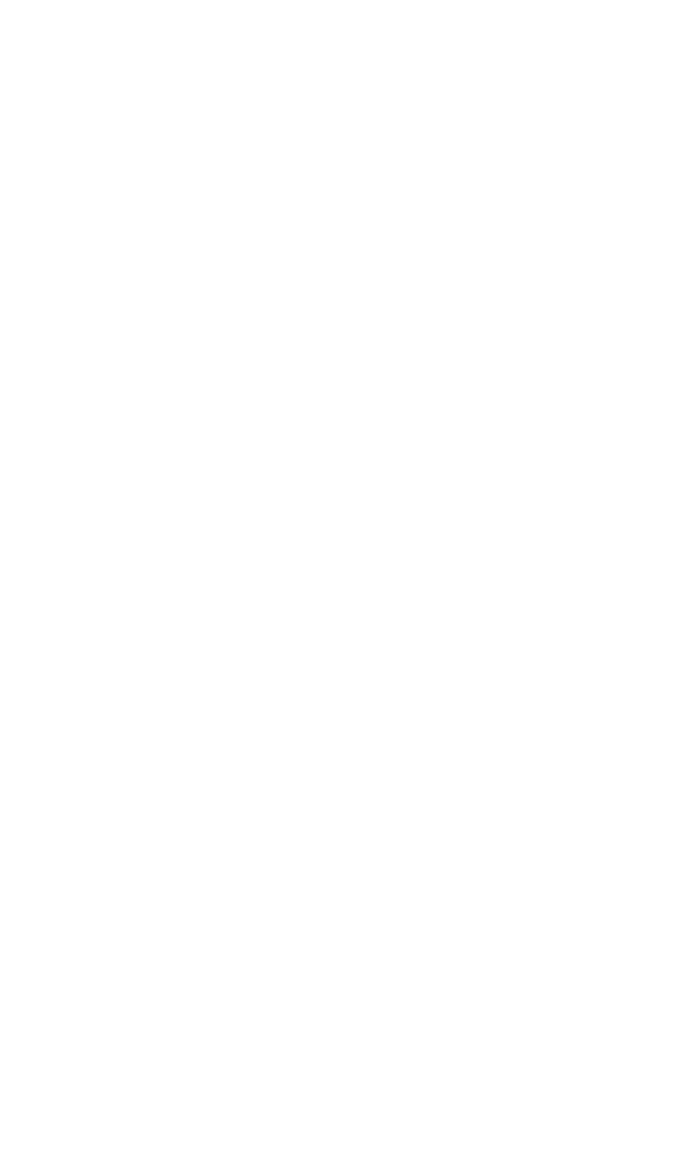
Search WWH ::

Custom Search