Environmental Engineering Reference
In-Depth Information
3. Provide an initial guess for w or update it from the prior calculation.
4. Using a NASTRAN complex eigenvalue solution procedure, compute modes, fre-
quencies and damping coefficients.
5. Select a mode with a small or negative damping coefficient and return to step 3 with
corresponding frequency update.
6. When the prior updated frequency is sufficiently close to the subsequently com-
puted one, either suspend computations or modify W and return to step 1.
The end goal in this process is to identify the eigenmode that exhibits a negative damp-
ing coefficient for the lowest rotor rotational speed. This speed is designated as the
classical
flutter speed
for the blade. A comprehensive approach would be to sequentially investigate
the stability of each mode by choosing its frequency as the initial guess for w, and tracking
that frequency over a range of increasing W. The flutter speed would then correspond to the
lowest value of W where the damping becomes negative over the set of modes investigated.
In practice, however, the first torsional blade mode produces the lowest values of W, coupling
with other blade modes (primarily flapwise modes) as the rotational speed increases. Of
course it is always prudent to check other modes that appear suspicious during the course of
the analysis.
Sample Flutter Calculation
After developing some confidence in the above computational procedure by comparing
its results to those of Theodorsen, it was applied to the large relatively soft wind turbine blade
shown in the wire-frame illustration of Figure 11-16. This blade, termed the baseline blade,
was designed as part of the WindPACT Rotor Design Study
[Malcolm and Hansen 2002].
Some characteristics of this blade and the associated rotor are listed in Table 11-5.
Figure 11-16. Wire-frame illustration of the 1.5 MW baseline WindPACT blade.
[Malcom and Hansen 2002]
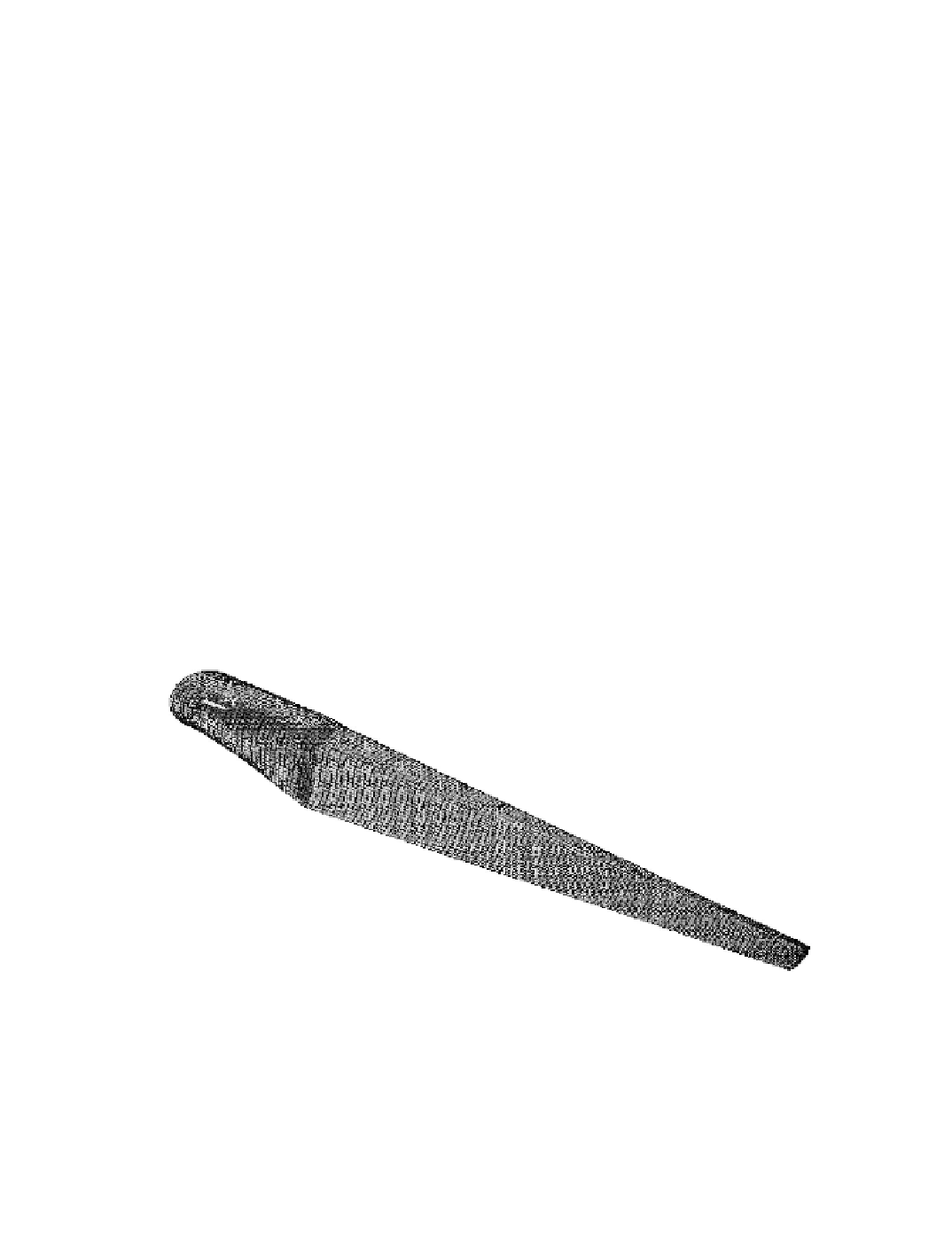
Search WWH ::

Custom Search