Environmental Engineering Reference
In-Depth Information
Development of Wind Turbine Wake Models
The earliest work on modeling wake interference appears to be by Templin [1974].
This was followed by a paper by Craaford [1975], who handled the subject by considering
the effect of the turbines as equivalent to a
ground surface roughness
and estimating the
flow retardation in the boundary layer caused by this effect. This approach gives a power-
ful overview with interesting global results, but it does not provide enough detail of the
wake structure for use in the practical design of wind power stations.
There are at least three general types of wake models, which are described in detail
by Luken and Vermeulen [1986]. The first is the
semi-empirical type
[Lissaman and Bate
1976]. Here a simple analytical relation is used to establish an invariant for the
fundamental wake scales of speed and radius. Experimental data are used for modeling the
flow profiles in various regions of the wake, and an empirical wake growth rate is used that
depends on turbulence. The second type of model [Ainslie 1985] utilizes an axisymmetric
boundary-layer
approximation of the Navier-Stokes equations
,
with an eddy viscosity model
to determine shear stress. Finally, a
full mixing length/eddy viscosity
(K,E) model [Crespo
et al.
1985] is an advanced type that takes into account surface roughness as well as atmo-
spheric stability. While this last procedure is valuable for analytical research, it is too
complicated for design purposes. Various approximations are employed in all three types of
models to reduce computer demands.
At the other end of the spectrum from the K,E model is an extremely simple analysis
developed by Katic
et al.
[1986]. In spite of its simplicity, wind tunnel tests of a cluster
of 37 model turbines indicate that its predictions are as accurate as those made with more-
complex models. As noted by these authors, good correlation between model predictions
and array test data is a consequence of satisfying the
conservation of wake momentum
,
which is the dominant invariant. Overall, a semi-empirical model contains practical
manifestations of all the significant fluid mechanic phenomena. Therefore, the basic prin-
ciples of wake modeling will be discussed here using the semi-empirical approach. Wake
models developed after 1994 are not covered.
Fluid-Dynamic Principles
Considering the turbine to be an
actuator disk
,
we note that it essentially removes
total
head
from the wind stream in the form of a pressure drop across the rotor disk. Because
there is no means of establishing the strong flow curvature required to maintain a pressure
difference between the wake and the surrounding free stream, the flow returns very rapidly
to the ambient static pressure. As a consequence, the loss of head is manifested in a
reduction in speed a short distance downwind of the disk. This is the so-called
velocity
deficit.
Standard turbine theory operates under the implied assumption that this deficit
extends unchanged from the disk to infinitely far downwind, which is known as the
Trefftz
plane concept
(Fig. 5-7). This concept is not adequate for determining flow details in the
wake itself, so we will construct a scheme for modeling the wake development downwind.
First, we define the wake as that portion of the flow that has a total head different from
the free stream. Because the wake velocity deficit exists within the free stream, there is the
characteristic axisymmetric
shear layer
between them, with turbulent transfer tending to
reduce velocity differences across the layer. As a consequence, the more-energetic free
stream is entrained into the wake. This increases both the mass flow and the total energy
in the wake. The net result is an asymptotic return of the wake flow to the undisturbed
conditions. However, since the flow in the wake just downwind of the rotor was of lower
energy, this return to the free stream state can occur only when the mass of air that passed
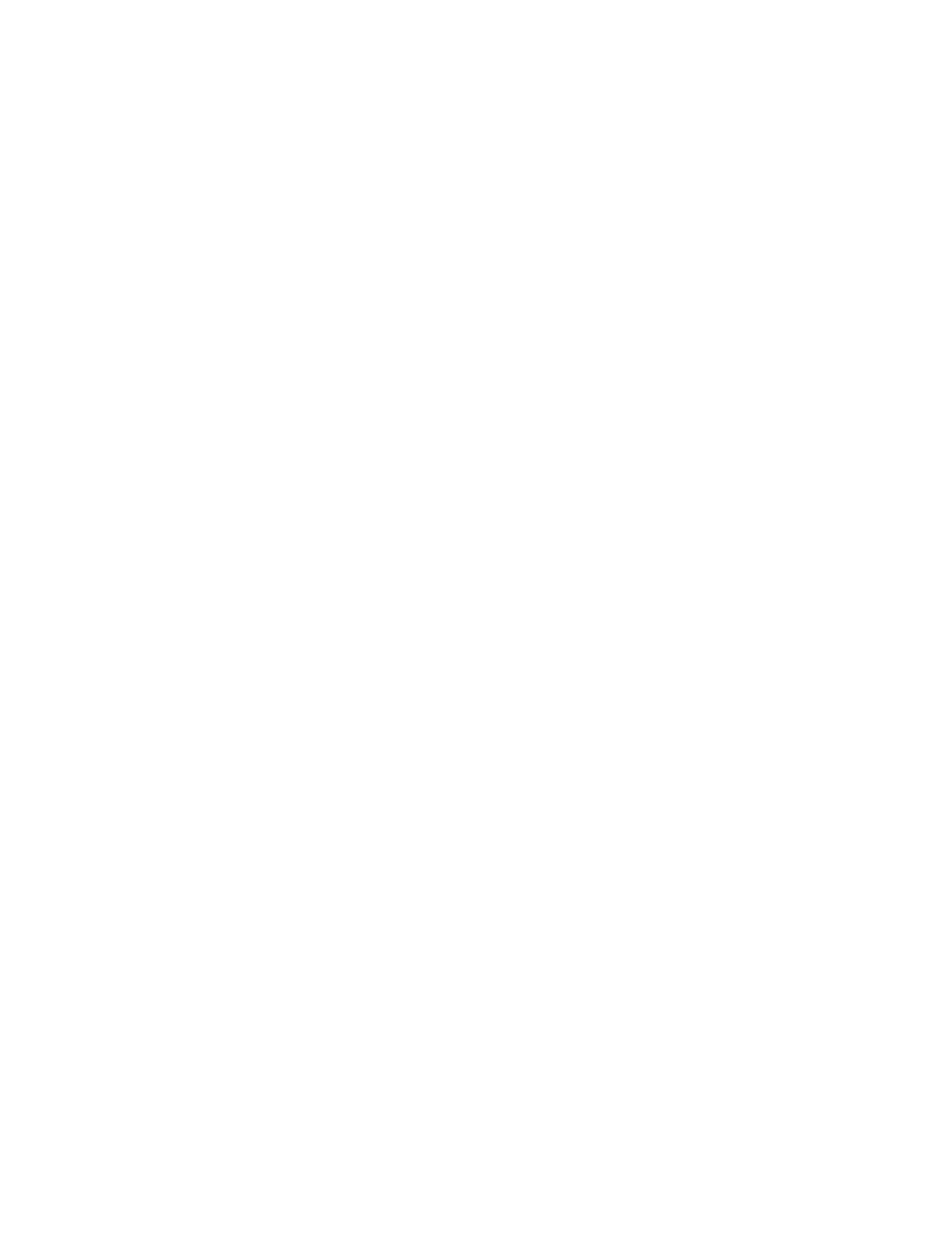
Search WWH ::

Custom Search