Environmental Engineering Reference
In-Depth Information
The energy balance can also be formulated in microscopic form, by returning to
the approach indicated by Equation (3.2), recognizing here that the energy density
f
=
v
2
, being the sum of the total internal energy density and the KE density
of the flow. PE is treated as source term, like radiation; thus,
s
f
=
ρ
u+½
ρ
!
+
Q
r
. Heat
transfer by radiation is dealt with in more details in Chapter 4. Note that chemical
reactions are no source of energy for the flow, as they only convert chemical energy
into thermal energy of the flow. The energy flux is now given by
!
ρ
+
p
!
!
+j
!
u+
1
2
ϕ
!
=
!
v
2
ρ
ρ
ρ
−
τ
ð
Eq
:
3
:
32
Þ
in which the first term represents the convective flux of internal energy and KE and
the other three terms arework exerted by pressure and viscous forces and the energy flux
due to conduction, respectively. Substitution of these terms in Equation (3.2) yields
+
+
+
∂
∂
j
!
=
u+
1
2
u+
1
2
v
2
!
v
2
p
!
!
!
!
+
Q
r
t
ρ
ρ
r
ρ
ρ
r
−
τ
r
ρ
ð
Eq
:
3
:
33
Þ
Setting up and working out energy balances is useful to describe the performance of a
system in terms of first law efficiencies. The concept of exergy makes use of the second
law of thermodynamics and enables valuing heat streams in terms of how much work
can actually be derived from them. Exergy is not conserved but is destroyed to a greater
or lesser extent by irreversibilities in the system. Although an exergy analysis adds value
to the analysis of the system performance with respect to energy conversion, this is con-
sidered to be out of the scope of this topic. We refer the interested reader to textbooks
such as Moran and Shapiro (2010), Çengel and Bolnes (2010), and Szargut (2005).
Example 3.3 Energy balance calculation (macroscopic): A continuous
pyrolysis vapor condenser
A biomass pyrolysis reactor produces a pyrolysis vapor, which is a complex mix-
ture of organic compounds and water. In this example, we consider an indirect-
contact heat exchanger for complete condensation of this vapor, with water used
as cooling liquid. The vapor (containing no liquid droplets) enters the condenser at
its boiling point of 380 K (assumed to be constant); it leaves the heat exchanger at
45
C. The cooling water is heated up from 20 to 35
C. The heat capacity of water
may be assumed to be constant at 4.18 kJ
K
−1
.
The heat of vaporization is assumed to be a typical value for an organic acid:
400 kJ
kg
−1
kg
−1
. The heat capacity at constant pressure, c
p
, is assumed to be constant
kg
−1
K
−1
.
at 2 kJ
a. Calculate the ratio of the mass flow rates of the condensing vapor and the
cooling water.
b. Calculate the rate of energy transfer from the condensing vapor to the cool-
ing water in kJ
kg
−1
of vapor passing through the condenser.
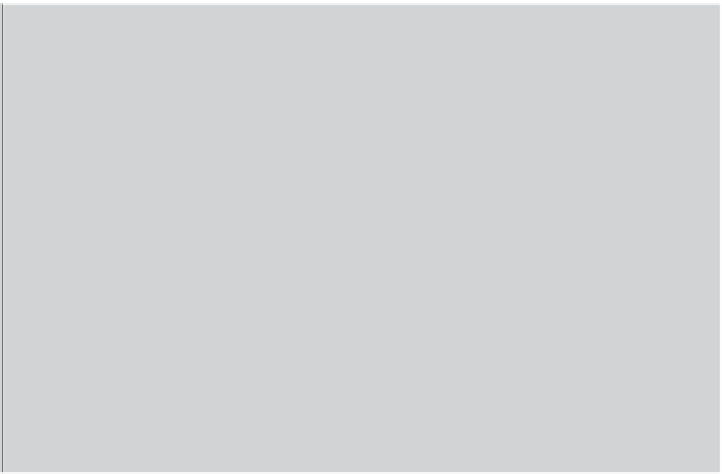
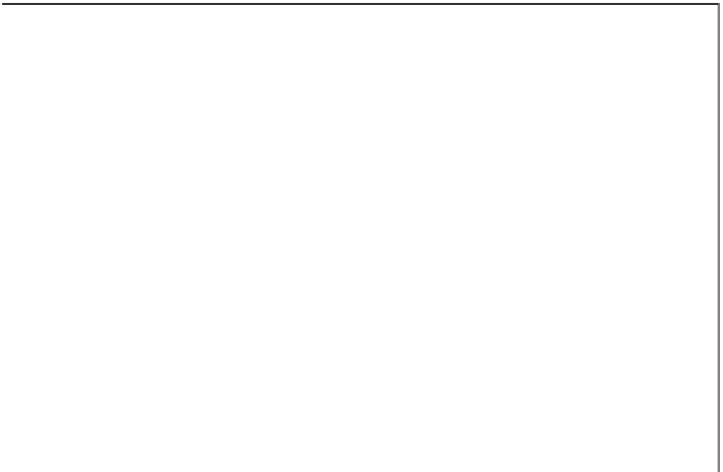










Search WWH ::

Custom Search