Geology Reference
In-Depth Information
Box 10.4 How to derive the isochron equation
Substituting
87
Rb
t
The kinetics of the Rb-Sr isotope system was briefly intro-
duced in Box 3.2. The general decay equation may be writ-
ten (equation 3.2.3):
e
λ
in place of
87
Rb
0
in Equation 10.4.3,
Rb
t
we get:
(
)
87
87
t
87
87
t
Sr
=
Rb
e
λ
−
Rb
=
Rb
e
λ
−
1
(10.4.5)
Rb
Rb
t
t
t
t
P
=
()
−
0
nne
P
λ
t
(10.4.1)
P
Equation 10.4.5 represents a curve similar to Figure 3.1b.
This equation tells us only the amount of
radiogenic
87
Sr
that has formed in the sample since
t
= 0. Because
87
Sr is
a naturally occurring stable nuclide, we need to allow for
the
87
Sr that was already present in the sample at
t
= 0
(see Figure 10.1b). Calling the amount of
87
Sr initially pre-
sent
87
Sr
0
, the
total
87
Sr present in the sample at time
t
is:
where
n
P
is the number of parent (radionuclide) nuclei pre-
sent in the sample at time
t
, (
n
P
)
0
is the number that were
initially present (at
t
= 0),
λ
P
is the decay constant for the
decay of the parent radionuclide, and
t
is the time elapsed
since the event being dated. Writing this in a more reader-
friendly style specific to the decay of
87
Rb:
87
87
Rb
t
(10.4.2)
Rb
=
Rb
e
−λ
(
)
87
87
87
t
Sr
=
Sr
+
Rb
e
λ
−
1
t
0
(10.4.6)
Rb
t
0
t
Here
87
Rb
t
represents the number of
87
Rb nuclei present at
time
t,
and so on. Equation 10.4.2 represents a curve simi-
lar to Figure 3.1a.
Every
87
Rb nucleus that decays generates a new
87
Sr
nucleus in its place. The number of radiogenic
87
Sr nuclei
accumulating in time
t
is equal to the number of Rb nuclei
that have decayed, which can be equated with the number
of
87
Rb nuclei initially present
minus
the number remaining
at time
t
:
In terms of practical laboratory analysis, it is much easier to
measure isotope
ratios
in a mass spectrometer than abs-
olute amounts of individual nuclides. Therefore dividing each
term in this equation by the amount of a reference stable
isotope
86
Sr, we arrive at a much more useful equation -
called the
isochron equation
- expressed in terms of easily
measured, present-day Sr isotope and Rb/Sr ratios:
87
86
Sr
Sr
=
87
86
Sr
Sr
+
87
86
Rb
Sr
(
)
t
(10.4.7)
e
λ
−
1
Rb
87
87
87
Sr
=
Rb
−
Rb
(10.4.3)
t
0
t
t
0
t
Since
87
Rb
0
is generally unknown in a geological dating
context, we eliminate it from Equation 10.4.3 by rearrang-
ing Equation 10.4.2 as follows:
87
86
Sr
Sr
Here
is the sample's current Sr isotope ratio
t
87
86
Rb
Sr
measured by mass spectrometry.
is calculated
t
87
87
from the ratio of the sample's Rb and Sr element concen-
trations (determined by routine analytical methods), and
λ
Rb
is the relevant decay constant (Table 10.1).
Rb
Rb
Rb
Rb
Rb
1
t
= ∴ =
e
−
λ
t
0
Rb
(10.4.4)
87
87
e
−
λ
t
Rb
0
t
λ
t
87
87
λ
t
=∴ =
e
Rb
e
Rb
Rb
0
t
Figure 10.5 shows a published isochron for a
Proterozoic intrusive complex, to illustrate the prin-
ciples explained here. Note how the various intrusive
units defined by field mapping differ in Rb and Sr con-
tents and in
87
Rb/
86
Sr ratio. Accurate age determination
requires the analysis of samples covering a significant
range of Rb/Sr composition.
The samples used in Figure 10.5 were whole-rock
samples. Another way to secure cogenetic samples with
a range of Rb/Sr ratios for accurate age determination
is to analyse mineral separates from a single rock sam-
ple, leading to what is called a
mineral isochron
(see
Exercise 10.2). Each mineral, upon crystallizing, inher-
its the magma's
87
Sr/
86
Sr value but, owing to element
fractionation during crystallization, acquires a different
Rb/Sr ratio. Mineral isochrons are more susceptible to
resetting by later thermal re-equilibration, and can
therefore be used to date episodes of metamorphism.


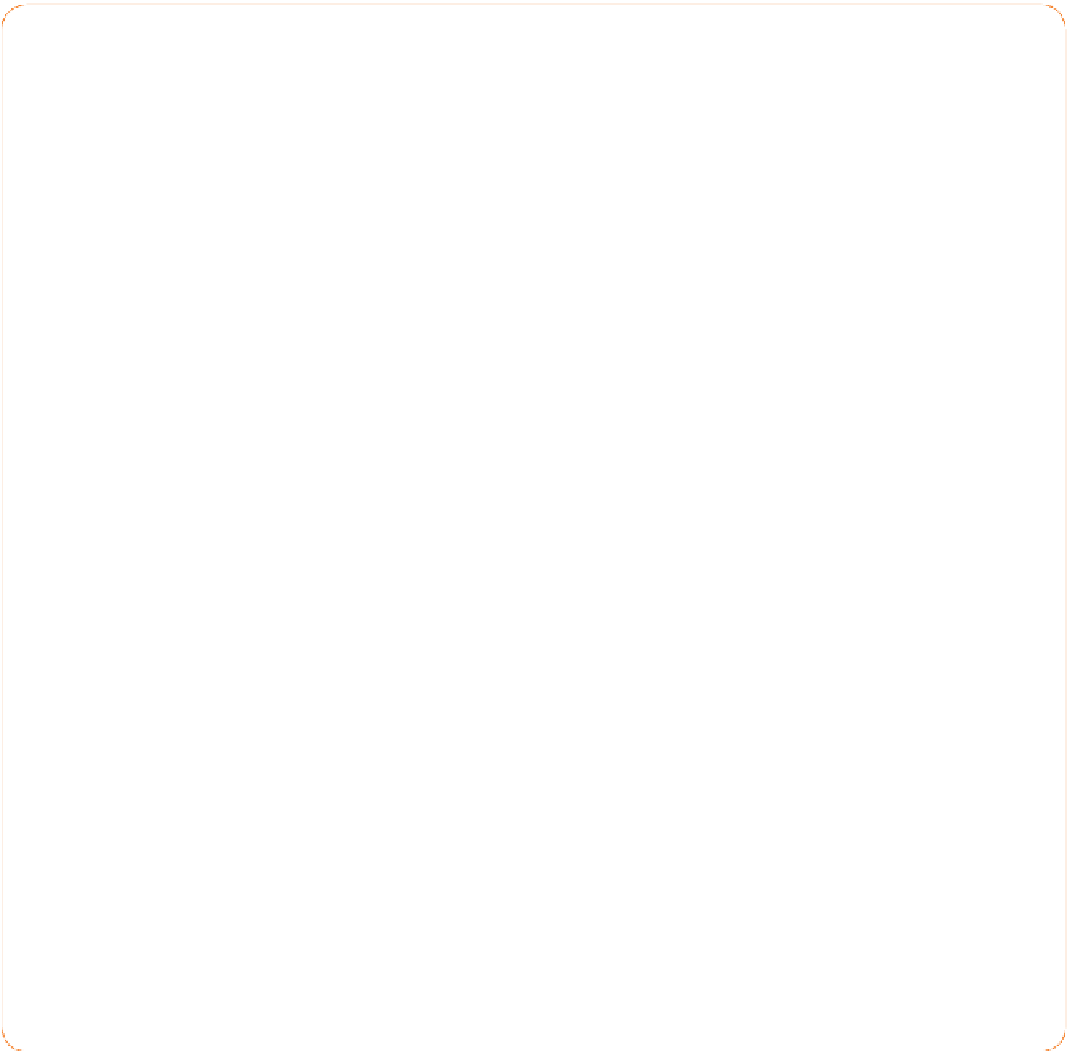


Search WWH ::

Custom Search