Geology Reference
In-Depth Information
Box 10.1 The nuclide chart
The clearest way to visualize the nuclides, both stable and
radioactive, is to plot their
Z
values against
N
values as
shown in Figure 10.1.1 (of which Figure 10.1a forms a
small part).
The
stable
nuclides (solid circles) lie in a narrow, slightly
curved ribbon. The most abundant light nuclides have
N ≈ Z,
but the stable isotopes of heavier elements become
increasingly more neutron-rich (
N
≤ 1.5
Z
). On each side of
the stable-nuclide ribbon lies a band of radioactive
nuclides, most of which are too short-lived to be found in
nature (so are not shown individually in Figure 10.1.1).
Neutron-rich (high-
N
) radionuclides lying to the right of the
stable-nuclide ribbon decay by ejecting a high-energy elec-
tron (for historical reasons electrons emitted by nuclei are
called
β
-particles, and being negatively charged are sym-
bolized
β
−
):
A positron (
β
+
) is the antimatter particle equivalent to
the electron, but with a positive charge. Its emission
signals a reaction in the potassium nucleus by which a
proton is transformed into a neutron
(Z
→
Z
− 1
,
N
→
N + 1).
The same transformation can also be accomplished by
capturing
an orbital electron. In either case the decay
appears in the nuclide chart as a short 'down-to-the-
right' transverse arrow (see decay of
40
K in the inset in
Figure 10.1.1).
Both
β
−
-decay and
β
+
-decay are isobaric reactions,
leaving
A
unchanged. Isobars - nuclides sharing the
same mass number or
A
-value - lie on diagonal lines in
Z-N
space - see the dashed line in the Sm-Nd inset in
Figure 10.1.1.
The third category of nuclear decay shown in Figure 10.1.1
is the ejection of an alpha-particle
α
2+
(consisting of 2
protons + 2 neutrons =
4
He nucleus):
87
87
Rb
→+
Sr
β
+
υ
neutrino
(10.1.1)
parent
nuclide
anti
daughter
nuclide
beta
particle
147
143
2
Sm
N
→+
α
+
Loss of the
β
-particle transforms one neutron in the
nucleus into a proton, increasing
Z
by one at the expense
of
N
. Such decays therefore appear in Figure 10.1.1 as
short 'up-to-the-left' transverse arrows, as illustrated by
87
Rb (see inset).
Proton-rich radionuclides on the left of the stable-nuclide
ribbon decay by ejecting a positron:
Such
α
-decay reactions reduce both
Z
and
N
by 2 and
A
by
4, so appear in Figure 10.1.1 as a trend-parallel arrow
pointing down to the left (see Sm-Nd inset). The compli-
cated decays of uranium (
238
U and
235
U) and thorium (
232
Th)
to different isotopes of lead (
206
Pb,
207
Pb,
208
Pb) involve
multiple
α
and
β
−
decay steps (Box 3.3).
87
Rb,
40
K,
147
Sm,
232
Th,
235
U and
238
U are all long-lived,
naturally occurring radionuclides which are used exten-
sively in geochronology.
40
40
K
→+
Ar
β
+
υ
(10.1.2)
parent
daughter
positron
neutrino
while
87
Sr increases with time in relation to other Sr
isotopes (Figure 10.1b). Radiogenic isotope systems
not only lie at the heart of geochronology, but also
shed important light on the provenance of igneous
magmas, metamorphic rocks and sediments.
Take care not to confuse
radiogenic
with
radioactive
.
16
O and
18
O (18 − 16 = 2) is relatively large in com-
parison to their mean mass number (17) - with
consequent slight differences in quantitative chemical
parameters - the
18
O/
16
O ratio is
fractionated
to a
measurable extent by geological processes such as
crystallization and hydrothermal alteration. The
minute natural variations that are seen in this ratio
between minerals and fluids therefore serve as a
useful
tracer
for detecting and quantifying such
processes.
Since their isotope ratios (e.g.
18
O/
16
O and
34
S/
32
S)
do not change with time, such systems are designated
stable isotope systems
.
Stable isotope systems
The three isotopes of oxygen (
16
O,
17
O and
18
O) are
neither radioactive nor radiogenic and so their rela-
tive proportions
do not vary with time
. Nonetheless,
because the difference in mass number
A
between
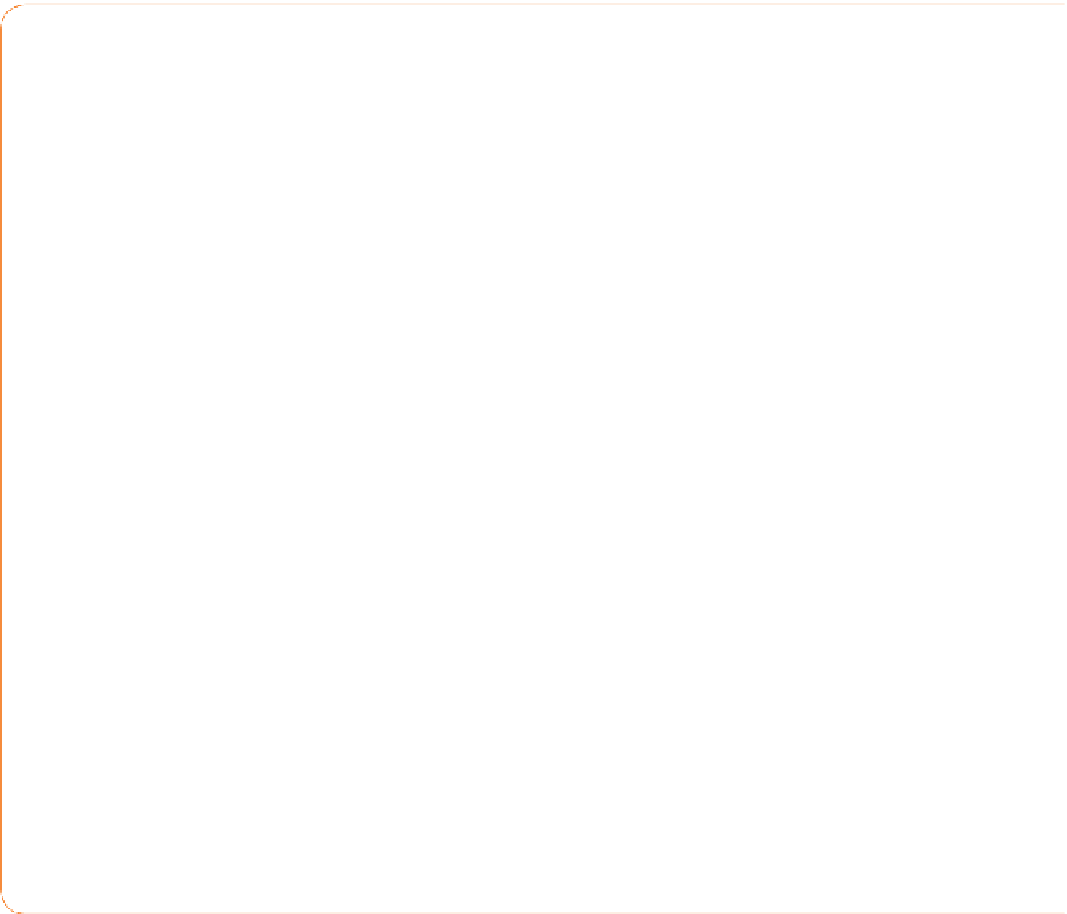

Search WWH ::

Custom Search