Geology Reference
In-Depth Information
Box 7.1 Equilibrium internuclear distance
two oppositely charged ions (for example, Na
+
and Cl
-
) are
attracted towards each other by their opposite net charges
(±e), and the attraction increases as they get closer. the
potential energy of this system is:
Repulsion term
be
2
r
12
+
Net potential energy
p
=−
2
e
r
be
2
r
12
e
2
r
E
(7.1.1)
+
=-
as
r
- the distance between the two nuclei - gets
smaller, the potential energy becomes more negative, indi-
cating greater stability. When the ions get so close to
each other that the negative electron clouds begin to inter-
mingle, however, we have to allow for an element of repul-
sion in the energy equation:
r
0
E
p
r
o
Minimum
E
at
r
=
r
o
2
2
e
r
be
r
Attraction term
E
p
=−
+
(7.1.2)
12
e
2
r
-
long range
attraction
−
shortrange
repulsion
−
where
b
is a constant. the dashed curves in Figure 7.1.1
show the two terms plotted separately. the
r
12
term indic-
ates that repulsion is felt over a much shorter internuclear
distance than the attractive force, but rises very steeply as
r
is reduced below a critical value.
the solid curve in Figure 7.1.1 shows the result of add-
ing the two terms together (equation 7.1.2). the energy
minimum defines an equilibrium internuclear distance or
'bond-length'
r
0
at which the isolated ion pair is most stable.
Figure 7.1.1
how the potential energy
E
p
of two oppos-
itely charged ions varies with internuclear distance
r
.
(When the cation is associated with more than one anion,
as in a crystal, repulsion between the anions makes
r
o
somewhat larger.) the rapid rise of the curve to the left of
this minimum reflects the observation that the ions strongly
resist getting closer together, rather like hard rubber balls.
that may mean), but an empirical 'radius of approach'
governing how close to it another ion can be placed.
X-ray diffraction data (Box 5.3) provide accurate equi-
librium bond lengths for many binary (two-element)
ionic salts like NaCl, NaF and CaF
2
. The problem in
determining the individual ionic radii of Na
+
and Cl
-
lies in deciding how much of the measured Na-Cl bond
length to attribute to each ion, the radius of neither
being known initially. An early approach to the prob-
lem, devised by the American Nobel Prize-winning
chemist Linus Pauling in 1927, was to examine com-
pounds like NaF in which the cation and anion happen
to have the same number of electrons (10 in this case).
Pauling suggested a relationship between ionic radius
and nuclear charge in such ion pairs which, together
with the measured Na-F bond length, allowed the sepa-
rate ionic radii to be estimated (see Fyfe, 1964, Chapter 4).
Modern ionic radii are based on more elaborate cal-
culations, although chemists are still not universally
agreed on the best values to use: published estimates
of the O
2-
radius, for example, vary between 127 and
140 pm (Henderson, 1982, Chapter 6). Mineralogists
and geochemists nonetheless find ionic radii extremely
useful in explaining the chemical make-up of crystal-
line materials. The ionic radii of some geologically
important elements are illustrated in Box 7.2.
One can see from Figure 7.2.1 that cation radii vary
considerably, from 34 pm (Si
4+
) to about 170 pm (Rb
+
).
Note the marked
decrease
in cation radius in proceeding
from left to right in the Periodic Table, in response to
increasing nuclear charge. Na
+
, Mg
2+
, Al
3+
and Si
4+
each
possess ten electrons, but they are pulled closer to the
silicon nucleus (charge 14+) than to the sodium nucleus
(11+). Anion radii are larger, owing to the extra elec-
trons they have acquired and to the mutual repulsion
between them. O
2-
, F
-
, S
2-
and Cl
-
are larger than all
cations except those of the alkali metals and the heavier
alkaline earth elements (Figure 7.2.1). Consequently

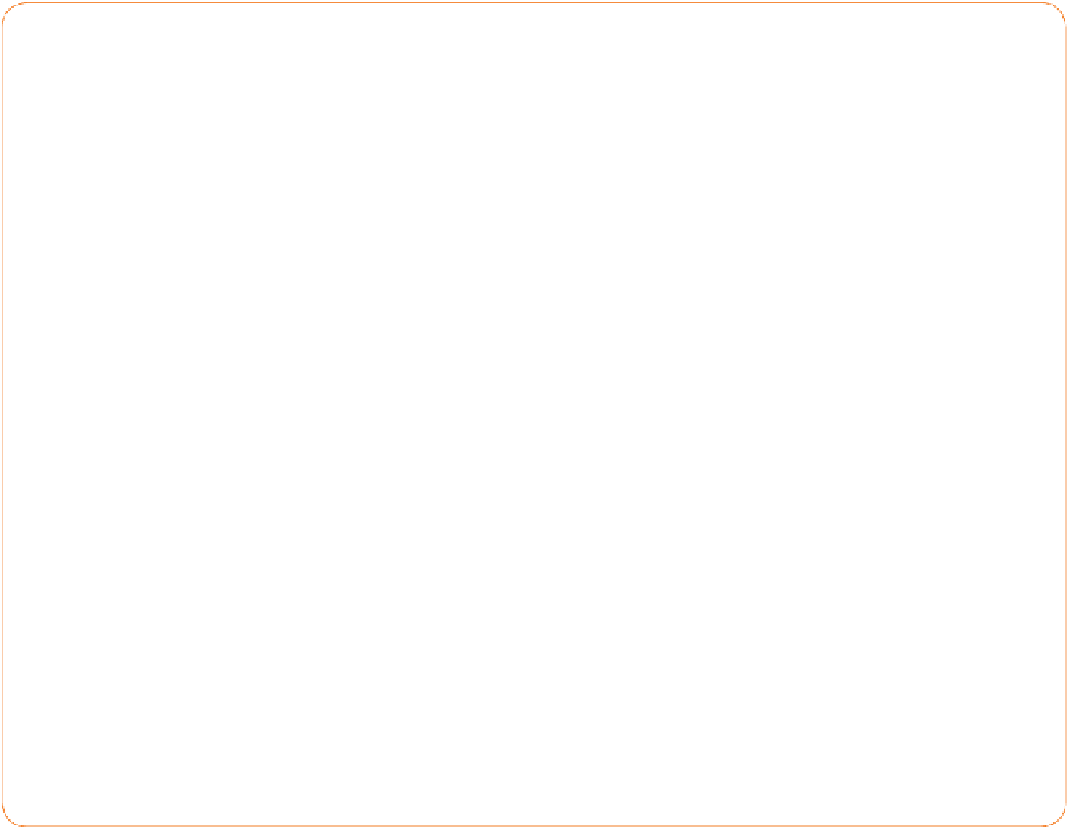




















Search WWH ::

Custom Search