Chemistry Reference
In-Depth Information
(a)
(b)
(c)
(d)
(e)
(f)
fIgure 14.5
Schematic representation of loading strategies of drugs and genes on microbubbles. (a) Non-covalent binding of DNA to the
surface of cationic lipid microbubbles. (b) Multilayered structure based on a lipid microbubble sequentially coated with DNA and poly-l-lysine
layers. (c) Polymeric microbubble loaded with hydrophobic drug loaded in the shell phase. (d) Polymeric microbubble with hydrophilic drug
loaded in the internal void. (e) Internal structure of polymeric microbubbles: Water-phase is dispersed through the polymer matrix, forming
upon lyophilisation a plurality of cavities distributed over the particle volume. (f) Attachment of liposomes or nanoparticles to the surface of
microbubbles through biotin-avidin-biotin bridging system. (Reproduced from Ref. [36], with permission from Elsevier).
sonoporation can be accomplished by applying transcranial US with intravenous injection of microbubbles without dam-
aging the neurons [91]. Delivery of both low and high molecular weight therapeutic compounds to the central nervous
system can be potentially attained through this noninvasive approach. Microbubbles are also used to enhance the noninvasive
high intensity-focused US therapy by increasing the local heating rate [92].
14.3.5
future applications
The potential of molecular imaging and therapeutic interventions can be compromised by the limited sensitivities. Advanced
microbubble fabrication technology could be sought to optimise size distribution, microbubble shell structure, ligand attach-
ment, and drug/gene incorporation. Efforts could also be made in advancing US beam technique in order not to interfere with
microbubbles.
14.4
aPPlIcatIons In MagnetIc resonance IMagIng
Relying on the differential decay and recovery characteristics of the nuclear magnetic resonance signals, magnetic resonance
imaging (MRI) provides superb soft tissue contrast with high spatial resolution when compared with other imaging modal-
ities. While MR image contrast can be flexibly controlled by varying pulse sequences and parameters, it is determined by
the intrinsic tissue properties such as proton density, longitudinal relaxation time (
T
1
), and transverse relaxation time (
T
2
). At
present, the exogenous contrast agents available for MRI mainly fall into three categories: gadolinium chelates, manganese
chelates, and superparamagnetic iron oxide particles. Their effects are usually described by longitudinal relaxation rate (
R
1
)
and transverse relaxation rate (
R
2
/
R
2
*
), where
R
1
,
R
2
and
R
2
*
are defined as 1/
T
1
, 1/
T
2
, and 1/
T
2
*
respectively. Susceptibility
contrast agents exhibit large
R
2
*
/
R
1
ratios and predominantly induce signal loss through spin dephasing by strong magnetic
susceptibility effects. Their
T
2
*
shortening effects are usually much stronger than the baseline
T
2
effects.
14.4.1
as an Mr susceptibility contrast agent
Microbubbles can potentially be used as an intravascular MR susceptibility contrast agent
in vivo
due to the induction of
large local magnetic susceptibility difference by the gas-liquid interface. Microbubble-induced signal perturbation depends
on the microbubble radius, volume fraction, overall magnetic susceptibility difference between the microbubble and the
blood plasma, and amplitude of the static field [93, 94].
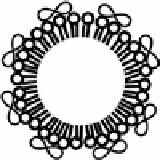
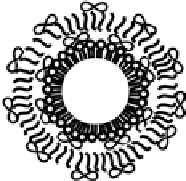
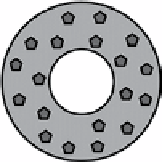
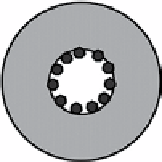
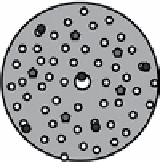
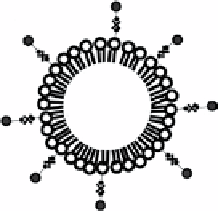