Chemistry Reference
In-Depth Information
(a)
(b)
Hydrogen nuclei
B
0
(c)
(d)
B
rf
B
0
B
0
FIgure 1.15 (
a) Hydrogen nuclei are randomly aligned in the absence of any strong magnetic fields. (b) All hydrogen nuclei, in the
presence of a strong magnetic field B
0
, are aligned in parallel with the magnetic field to create a net magnetic moment,
M
, which is parallel
to B
0
. (c) A radio-frequency pulse B
rf
is applied perpendicularly to the magnetic field B
0
. This pulse, with a frequency equal to the Larmor
frequency, causes the net magnetic moment of the nuclei M to tilt away from B
0
. (d) The rF pulse stops and the net momentum of the
nuclei realigns back in parallel to B
0
by relaxation, at the same time the nuclei lose energy to give an rF signal.
with B
0.
There are two different types of relaxation, T
1
and T
2
, the longitudinal relaxation time and the transverse relaxa-
tion time respectively. The T
1
and T
2
relaxation times define the way the protons revert back to their resting states after
the initial rF pulse. As protons relax, they realign along B
0
by T
1
where T
1
is the recovery of magnetisation along the
longitudinal axis. They also lose phase coherence by T
2
, which is the decay of magnetisation along the transverse axis.
These two parameters are the most significant in providing image contrast in MrI. The time constant of T
1
is tissue-
dependent. Signal strength decreases in time with a loss of phase coherence of the spins. This decrease occurs at a time
constant T
2
, which is always less than T
1
(Figure 1.16). During T
1
and T
2
relaxation, the nuclei lose energy by emitting
their own rF signals; however, only transverse magnetisation produces a signal. This signal is referred to as the free-
induction decay (FID) response signal. The FID response signal is measured by a conductive field coil placed around the
object being imaged, and the FID decays at a rate given by the tissue relaxation parameter known as T
2
*. The measurement
of the FID signal gives images that have different weightings depending on the T
1
, T
2
, and T
2
*. These signals are pro-
cessed or reconstructed to obtain greyscale contrast 3D images [24]. The different properties between T
1
and T
2
are shown
in Table 1.2.
Although the human body contains a high percentage of water, the signal intensity is not just dependent on the amount of
water at the location, and experimentally it is hard to change the proton density in tissue to look at small changes. Thus,
chemical contrast agents are used to change the characteristics of tissue by altering the magnetic relaxation times of T
1
and
T
2
, which normally amplifies the contrast, and these agents are classed as T
1
and T
2
agents.
Ferromagnetic contrast agents alter the contrast by changing the T
2
* of the water molecules around the ferromagnetic
contrast agent by distorting the B
o
magnetic field around the ferromagnetic material in the contrast agent. These contrast
agents are typically iron nanoparticles with bio-organic compatible substrates. Paramagnetic contrast agents, which are
much more commonly used, alter the contrast by producing time-varying magnetic fields that promote T
1
relaxation of water
molecules. The time-varying magnetic fields come from both rotational motion of the contrast agent and electron spin flips
associated with the unpaired electrons in the paramagnetic material in the contrast agent. This is why gadolinium agents are
the most favourable because the f-element Gd ion has the maximum seven unpaired electrons [29].














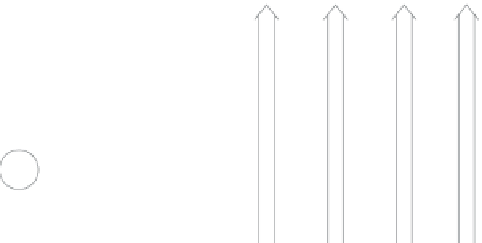











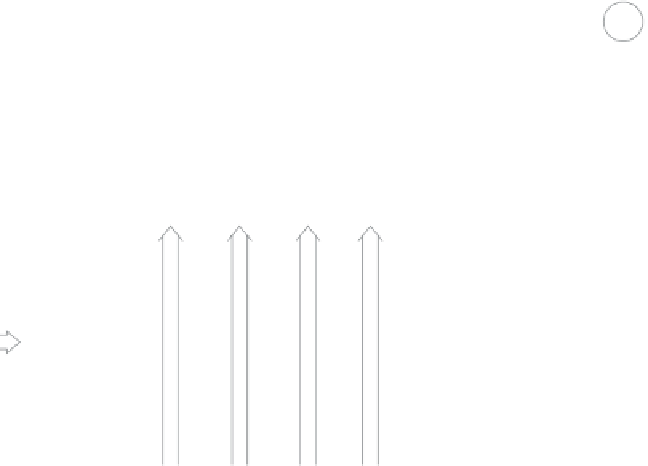















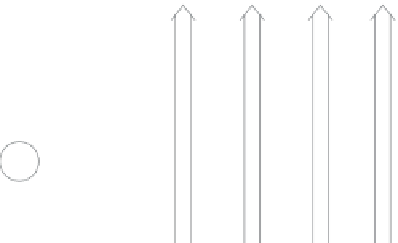
















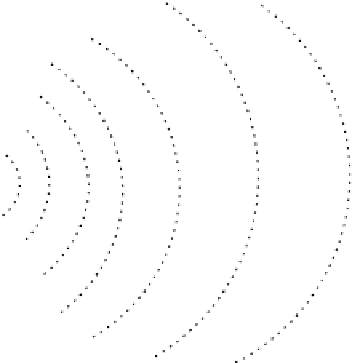




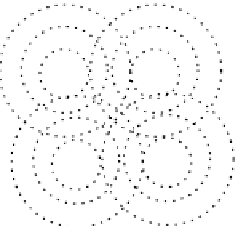








