Biomedical Engineering Reference
In-Depth Information
and tissue engineers have yet to match Nature's
success. In fi rst approaching the problem, it is
helpful to consider the critical functions that
the engineered tissue needs to replace. Bone, as
designed by Nature, is a remarkably resilient
and multifunctional, dynamic, and self-healing
structure. Bone is one of the few tissues in the
human body that heals without scarring, and it
represents as such an ultimate smart material.
If one sets out to design the ultimate smart
material success may be elusive; however, if one
considers the mechanisms behind the remark-
able capacity of bone not to scar, it is possible
to gain insight into one of the most powerful
intrinsic material properties of bone, i.e., the
capacity to adapt its structure to its prevailing
function over time. It is not the material of
bone per se that imparts this remarkable prop-
erty to the tissue, but the cells within bone that
are the biological machines continually build-
ing and rebuilding structure in response to the
prevailing dynamic environment. Hence, any
rational tissue-engineering approach must
consider the cells and the fact that they migrate
within the dynamic environment of the tissue
in times of tissue modeling, homeostasis, and
disease.
The role of fl uids in bone tissue engineering
has been receiving increasing attention. In fact,
from the perspective of fl uids alone, bone is an
ecosystem. The transport of life-supporting
substances and the removal of waste are basic
requirements for the maintenance and survival
of any ecosystem. The human body and subsets
of the body, e.g., organ systems, are also eco-
systems with water, gases, nutrients, waste
products, and regulatory substances such as
hormones and cytokines in constant fl ux within
the system (Fig.
Figure 10.1.
Pieces of the “bone model puzzle.” Depending
on the system of interest, bone can be modeled in a variety of
ways. Key elements that are common to all models include the
function that is to be replaced (which defines the goal of the
model), the control volume (an abstract representation of the
highly idealized model that aids in reducing the system to one
with a finite, determinate set of variables), governing equa-
tions that provide mathematical predictions of model behavior
in response to changes in system variables, boundary condi-
tions, and initial conditions. The size and boundaries of the
model system or control volume are determined by a variety of
factors, including the tissue type and component to be
modeled, as well as the length and time scale to be addressed
in the system of interest. ECM (extracellular matrix).
problem can be approached at multiple length
and time scales
. The chapter is not designed as
a cookbook for computer modeling; rather it
is intended to encourage tissue engineers to
utilize modeling, thereby increasing the power
of their research.
). Different hierarchical
levels defi ne organ, tissue, and cell physiology
within the entire system. Materials are trans-
ported into these mostly in the form of water
solutes. Except for the case of respiratory gas
exchange with the external environment, fl uid
convection represents the most powerful trans-
port mechanism throughout the human body
[
10
.
2
10.2 Nature's Design
Solution: the Biological
Ecosystem cum Gold Standard
for Tissue Design
Specifications
].
By considering the mechanisms that
underlie specifi c functions of bone, tissue
engineers can make use of specifi c aspects of
the structure of bone when designing tissue
replacements. The functions of bone are many.
At a systems level, the skeleton provides
mechanical support, making it possible for a
18
] and in bone in particular [
8
Nature's designs are full of complexities and
redundancies, yet designing a multifunctional
tissue such as bone is a technical tour de force,
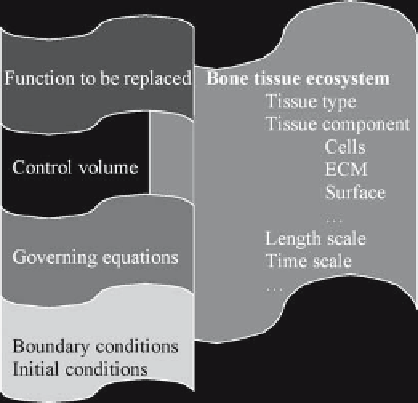