Biomedical Engineering Reference
In-Depth Information
8.4 Micromotion and Implant
Osseointegration
with soft tissue, thus decreasing element stiff-
ness. Here, the MES was computed locally in
10
-thick regions of osseointegrated tissues
around individual beads over a whole implant
interface. Homogenization theory [
-
µε
45
,
53
,
67
,
8.4.1 Orthopedic Porous Implants
69
] was used to calculate local strains by cou-
pling the local microstructural model with the
global model.
Parametrical analyses by varying loading
directions, osseointegrated tissue modulus (E
=
0
We have developed a series of two-dimensional
fi nite element models to calculate interfacial
tissue strains inside the pores of porous coated
implants, based upon analyses of histological
sections obtained from canine tibia [8,
.
01
,
0
.
1
,
0
.
5
,
1
,
5
, and
10
GPa), and levels of
]. We
hypothesized that long-term bone ingrowth to
the porous interface is related to the state of
local interface tissue strain. This hypothesis
was tested by comparing predicted values of
the amount of local bone (Ip) in the interfacial
zone with actual ingrowth (Ia) measurements
obtained from animal experiments [
67
MES (
)
were used to investigate the relationship
between motion and bone regeneration
(ingrowth). Linear regression between Ip and
the percentage of actual measured bone
ingrowth, Ia, was calculated. Coeffi cients of
determination,
r
, and the sum of squares dif-
ference, SSQD (
500
,
1000
,
1500
,
2000
,
2500
, and
3000
µε
|
2
), the accumulated dif-
ference between Ia and Ip, were then compared
by a three-way analysis of variancee (ANOVA)
(Prophet
], using
a rule relating ingrowth distribution to calcu-
lated tissue strain magnitude. The rule is based
on the assumption that bone adaptation of
osseointegrated tissues obeys the minimum
effective strain (MES) theory postulated by
Frost [
43
σ|
Ia-Ip
, BBN Co., MA). The optimal range
of MES for correlating tissue strain with the
osseointegrated tissue was determined accord-
ing to the statistical results.
The results (Table
5
.
0
]. If the local bone element is subjected
to a maximum shear strain greater than a given
threshold value (MES), it will undergo a main-
tenance process, resorption-formation equilib-
rium, in which the element retains the same
properties as defi ned in the original model.
Otherwise, the bone is resorbed and replaced
37
) showed that the best
(highest
r
) angle for predicting Ia was
8
.
3
. This
result is consistent with the primarily vertical
loads applied to the canine tibia during gait.
The best bone modulus (E) for prediction was
0
0
.
5
GPa, followed by
0
.
1
and
1
GPa; however,
Table 8.3.
Summary of the results of analyses with respect to correlation coefficient
r
(top) and SSQD (bottom)
Source
DF
Sum of squares
F
ratio
Prob
>
F
Loading angle
6
1.852062
44.5449
0.0000
Modulus (GPa)
5
10.974497
316.7441
0.0000
Loading angle* modulus
30
2.039731
9.8117
0.0000
MES
5
0.123563
3.5662
0.0045
Loading angle* MES
30
0.084647
0.4072
0.9974
Modulus * MES
25
0.796406
4.5971
0.0000
Source
DF
Sum of squares
F
ratio
Prob
>
F
Loading angle
6
9754948746
21.7949
0.0000
Modulus (GPa)
5
3.18913*10
11
855.0325
0.0000
Loading angle* modulus
30
6909422103
3.0875
0.0000
MES
5
7.33802*10
10
196.7383
0.0000
Loading angle* MES
30
1018415745
0.4551
0.9933
Modulus * MES
25
1.95474*10
10
10.4816
0.0000
The relationship between Ia and Ip varied significantly (
p
<
0.05) with loading angle, modulus, and minimum effective strain (MES).
Abbreviations: DF, degrees of freedom; SSQD, sum of squares difference.
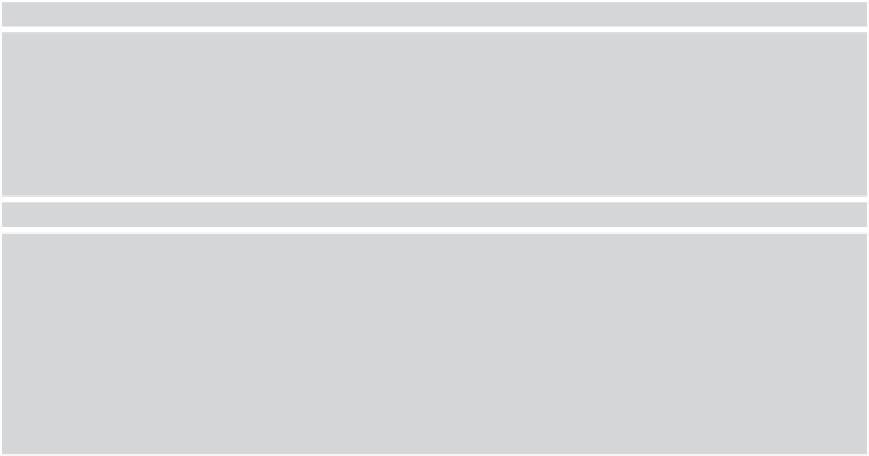