Geology Reference
In-Depth Information
30
(a)
(c)
(b)
±
1 Std dev.
Statistics:
N
= 151
Mean = 57.4
Median = 60.0
Std dev. = 24.0
Min. = 0
Max. = 90
25
Median
Vertical
Mean
20
15
10
5
0
10
Horizontal
0
20
30
40
50
60
70
80
90
>90
Vertical
(d)
(e)
Fracture angle class (deg)
(f)
Fig. 4.
Histogram of inclination angles for the whole
fracture interval in core Clino. The domination by
intermediate to vertical fractures suggests compressional
and perhaps some extensional forces are infl uencing the
fracture formation.
cementation between the fracture-host sediment
and the hemipelagic sediments is well illus-
trated in the downhole log signature (Fig. 7). The
cemented skeletal intervals tend to show a higher
sonic velocity relative to the background hemipela-
gic intervals. Similarly, the caliper log across the
fractured intervals shows borehole diameters
very close to the size of the diamond core bit,
whereas the hemipelagic units above, within and
below the cemented skeletal intervals show larger
diameters due to less cementation (Fig. 7). Within
the fracture intervals, both the sonic and caliper
logs show high-frequency variations, a signature
that probably refl ects the variability of fracture-
derived porosity and density.
The more intensely fractured rock in the
middle and upper intervals sometimes contain
secondary mineralization in the form of celestite
(Melim
et al
., 2001) (SrSO
4
also known as celes-
tine, Fig. 3f). The infi ll of fractures by celestite is
highly variable with respect to depth and aperture
size. In some cases, celestite has nearly fi lled the
fracture (see Fig. 3e), while in some of the larger
fractures elongate individual celestite crystal
are found lining the fracture surface (as in Fig. 3f).
The celestite infi ll clearly demonstrates the natural
origin of these fractures. The timing of the celestite
infi ll can be only poorly constrained. A celestite
crystal at ~386 m produced a Sr-isotope ratio with an
age range of 1.34-2.15 Ma. The uncertainty is high
for this age as the time of crystallization depends
on the two possible origins of the strontium: either
from dissolution of the aragonite or from seawater
entering the platform. Most likely, it is some
Fig. 3.
Photographs of fracture core. (a, b) through-going
shear fracture at 441 m depth; (c) axial fracture at 379 m,
note central fracture with small subfractures radiating
outward; (d) cataclastic shear fracture with a central
through-going shear, 431 m depth; (e) cataclastic fracture
at a bed contact at 437 m; celestite infi ll of larger fracture
openings, this photograph illustrates how minor differ-
ences in the degree of cementation probably affect fracture
potential; (f) fracture surface with large crystals of celestite
at 401 m depth.
broad scatter of values (Fig. 5). It is interesting
to note that the two intervening hemipelagic
intervals between the three fracture intervals do
contain several fractures, and that these fractures
are very steep to vertical. Unlike the fracture inclina-
tion with depth, the cumulative fracture length
(total fracture length in centimetres per 3 m inter-
val) does show a generally higher fracture density
near the tops of the three intervals and a trend of
decrease downward (poorly defi ned) (Fig. 6).
Host rock lithology, log signature and
fracture fi ll
The two main fracture intervals are hosted by the
well-cemented skeletal grainstones and packstone
units, termed 'interruptions' and derived from
the shallow platform to the east. The differential

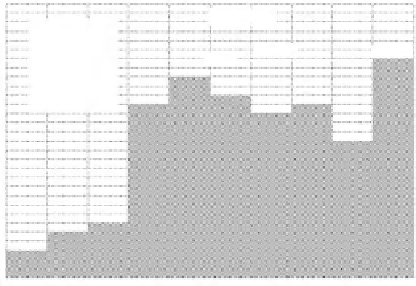





























































