Environmental Engineering Reference
In-Depth Information
where
K
is a dimensionless constant that can be taken
as equal t
o
0.04 in laboratory channels and 0.4 in natural
streams,
U
is the cross-sectional average longitudinal
velocity (LT
−1
),
B
is the top width of the channel (L),
and
r
c
is the radius of curvature of the stream (L). For
straight channels of any cross-sectional shape, Deng
et al. (2001) proposed that ε
t
be estimated as
and the corresponding downstream length scale,
L
w
(L),
to where the tracer is well mixed over the width can be
estimated by
2
Vw
L
=
VT
=
(4.14)
w
w
ε
t
Combining Equations (4.12) and (4.14) leads to
1.38
1
3520
U
u
B
d
ε
t
=
0.145
+
du
(4.10)
*
*
2
L
L
w
d
ε
ε
=
w
v
(4.15)
d
t
More complex expressions for ε
t
have also been pro-
posed that give the variation of ε
t
with distance along
the channel (e.g., Baek and Seo, 2011). Field experi-
ments have demonstrated that when formulations such
as Equations (4.7-4.10) are used to estimate ε
t
, more
accurate estimates are obtained by using channel prop-
erties (
d
,
u
*
) that characterize the portion of the channel
occupied by the tracer (Dow et al., 2009) and not the
cross-sectionally averaged values. Field experiments
have also shown that in northern streams, values of
ε
t
/
du
*
are approximately the same with and without ice
covered surfaces (Zhang and Zhu, 2011b). If a reliable
estimate of ε
t
is needed in any particular case, it is rec-
ommended that field experiments be conducted to
measure it directly (Roberts and Webster, 2002). overall,
typical values of ε
t
/
du
*
are 0.1-0.3 for relatively straight
channels, 0.3-0.9 for gently meandering channels, and
1-3 for sharp curved channels.
Many mixing-zone analyses assume instantaneous
cross-sectional mixing and then calculate longitudinal
variations in cross-sectionally averaged concentrations
downstream from the discharge location. Considering a
stream of characteristic depth
d
(L) and width
w
(L),
the time scale,
T
d
(T), for mixing over the depth of the
channel can be estimated by
According to Equations (4.6) and (4.8), it can reason-
ably be expected that in natural streams ε
t
≈ 10ε
v
, in
which case Equation (4.15) can be approximated by
2
L
L
w
d
w
(4.16)
=
0.1
d
Since channel widths in natural streams are usually
much greater than channel depths, typically width/depth
≥ 20 (Koussis and Rodríguez-Mirasol, 1998), for single-
port discharges, the downstream distance to where the
tracer becomes well mixed across the stream can be
expected to be at least an order of magnitude greater
than the distance to where the tracer becomes well
mixed over the depth. Therefore, mixing over the depth
occurs well in advance of mixing over the width. The
length scale to where the tracer can be expected to be
well mixed over the width is given by Equation (4.14).
The actual distance,
L
w
, for a single-port discharge
located on the side of a channel to mix completely
across a stream has been determined from field mea-
surements (Fischer et al., 1979) as
′
2
d
2
Vw
T
d
=
(4.11)
L
′ =
0.4
(4.17)
ε
v
w
ε
t
and the distance,
L
d
(L), downstream from the discharge
point to where complete mixing over the depth occurs
is given by
In estimating the coefficient in Equation (4.17),
com-
plete mixing
was defined as the condition in which the
tracer concentration is within 5% of its mean every-
where in the cross section. Equation (4.17) can be
applied at any discharge location in a stream, where
w
is taken as the width over which the contaminant is to
be mixed to achieve complete cross-sectional mixing.
For example, if a diffuser of length
L
is placed in the
center of a stream of width
W
, full cross-sectional mixing
occurs when the contaminant mixes over a width,
w
= (
W
−
L
)/2, and the downstream distance,
2
Vd
L VT
=
=
(4.12)
d
d
ε
v
Similarly, the time scale,
T
w
(T), for mixing over the
width,
w
, can be estimated by
2
w
L
w
, to
′
T
w
=
(4.13)
ε
t
complete cross-sectional mixing is given by



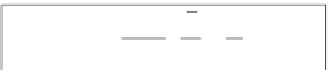



Search WWH ::

Custom Search