Environmental Engineering Reference
In-Depth Information
Figure 8.2 In situ SXS electrochemical cell: WE, working electrode; CE, counter-electrode;
RE, reference electrode. On the left is shown the transition from (1
1) to (hex) for a Au(100)
surface and on the right the characteristic adsorbate structures of CO on Pt(111) commonly
observed by SXS.
Fig. 8.2, takes advantage of the ability of X-rays to penetrate a thin solution
layer. Together with a simple theory of backscattering, this allows very detailed spatial
information to be obtained [Lucas and Markovic, 2006].
For instance, one of the most relevant systems to study is reconstruction of
Au(100), and its (hex)
$
(1
1) transition. This transition can be followed by SXS
[Ocko et al., 1990; Tidswell et al., 1993], as the reconstruction gives rise to X-ray
scattering that is separated in reciprocal space from the scattering due to the bulk
of the crystal. Figure 8.2 shows ball models of the square planar (1
1) surface
that is stable at positive potentials, and the hexagonal (hex) reconstructed phase,
which is commonly detected at more negative potentials. On the right-hand side in
Fig. 8.2, schematic structures of the Pt(111)-CO system are given. The potential-
induced p(2
2)
$
1
p
CO phase transition of an ordered CO adlayer has been
successfully followed by SXS in acid and alkaline electrolytes [Tolmachev et al.,
2004; Wang et al., 2005; Strmcnik et al., 2007]. Both examples in Fig. 8.2 are associ-
ated with a new SXS cell design, which allowed the exploration of temperature
effects on surface reconstructions of monometallic surfaces both in nonadsorbing
electrolytes and in the presence of different adsorbates, and for different electrolyte
pH values. Great progress has been achieved with SXS in studies of bimetallic
systems such as Pt
3
Sn(111) [Stamenkovic et al., 2003] and Pt
3
Ni(hkl)
[Stamenkovic et al., 2007a] surfaces, which will be discussed in more detail in the
following sections.
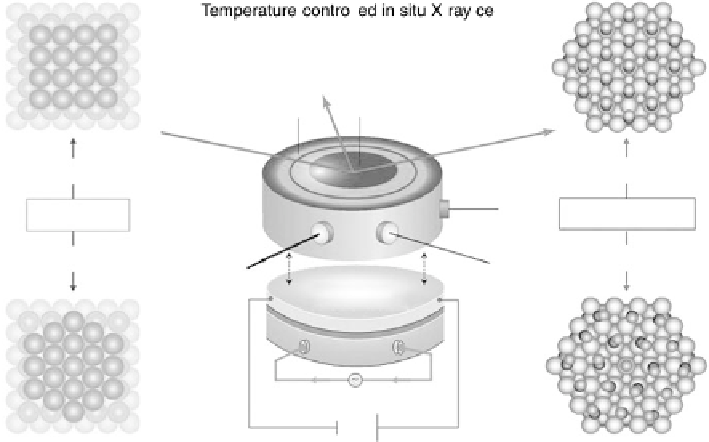











































Search WWH ::

Custom Search