Image Processing Reference
In-Depth Information
We can now define an “efficiency” factor as
C
G
Q
=
ext
(3.30)
ext
where
G
is the geometrical cross section.
Similarly, we can now define the corresponding efficiency factors
Q
sc
,
Q
abs
and
Q
ext
(
Q
ext
=
Q
sc
+
Q
abs
). Extinction from a single particle,
Q
ext
, can be very
large, especially at resonance. Defining
N
as the number of particles per unit
volume, we can express the relationship
Q
=
Q
(3.31)
ext
ext single particle
(
)
3.3.3 Mie Scattering
One very specialized and important scenario of the inverse scattering prob-
lem that will be looked at in detail later in this topic involves a target that is
an isotropic, homogeneous, dielectric sphere in 3-D or a disk in 2-D. This type
of problem is called a Lorenz-Mie scattering type, which was first published
in 1908. The basic setup with variable definitions is shown in Figure 3.3. In
general, Maxwell's equations are solved in spherical coordinates utilizing the
separation of variables method. The problem is solved for the case when the
field is determined at a distance that is much larger than the wavelength,
otherwise known as the far-field condition or zone. In the far field, the solu-
tion can be expressed in terms of two scattering functions as follows (van de
Hulst, 1957):
∞
∑
n
nn
21
1
+
+
S
()
Θ
=
)
[
a
π
(
cos
Θ
)
+
b
τ
(
cos
Θ
)]
(3.32)
1
(
nn
nn
n
=
1
∞
∑
n
nn
21
1
+
+
[
]
S
()
Θ
=
b
π
(
cos
Θ
)
+
a
τ
(
cos
Θ
)
(3.33)
2
(
)
nn
nn
n
=
1
X
S
scat
m
o
Φ
Θ
E
Z
S
inc
B
m
Y
Figure 3.3
Typical setup and coordinate geometry with variable definitions for the Lorenz-Mie scattering
problem.










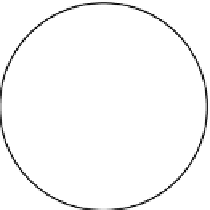














Search WWH ::

Custom Search