Geology Reference
In-Depth Information
(a)
(b)
(c)
(d)
(e)
(f)
(g)
(h)
Figure 7.18. Thermochemical plumes from a three-dimensional numerical model
[84] in which plume heads stall at the transition zone. Separation of more buoyant
material allows small columns to rise through the upper mantle. Copyright by the
American Geophysical Union.
melting can be explained if the plumes are 200-300
◦
C hotter than normal mantle,
and their longevity is plausible if they derive from a hot thermal boundary layer.
Their higher temperature implies that plumes would have lower viscosity than
normal mantle, and fluid dynamics experiments show that the preferred form of
low-viscosity buoyant upwellings is columnar, and that new plumes would start
with a large, spherical head. Plume heads are calculated to reach diameters of
about 1000 km near the top of the mantle, and they provide a plausible explanation
for flood basalt eruptions. The association of plume heads with their following
plume tails provides an explanation for hotspot tracks that emerge from flood
basalt provinces.
Plumes and the flow they drive in surrounding mantle comprise a distinct mode
of mantle convection, driven by a hot, lower thermal boundary layer. They are
estimated to carry about 3.5 TW of heat through the upper mantle and perhaps 7-
12 TW through the lowermost mantle, substantially less than the 30 TW estimated
to be carried by plates and much less than the total heat loss from the Earth of
about 44 TW. On the basis of this heat flow, and the topography from which it
is estimated, plumes thus seem to be distinctly secondary to plates as a mode
of mantle convection. Because they are driven by a hot, lower thermal boundary
layer, plumes are complementary to the plate mode, which is driven by the cool,
top thermal boundary layer. As with the plate mode, the upflow in plumes will be
balanced by a passive return flow, downwards in this case.
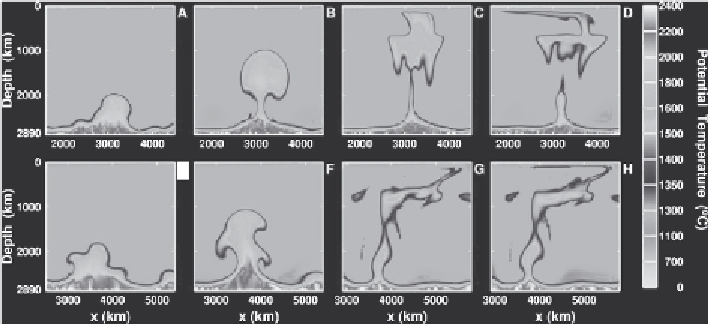





