Environmental Engineering Reference
In-Depth Information
oxide-oxygen interface to the oxide-metal interface as often experimentally
observed.
Of the three mechanisms proposed above to explain the role of active elements,
the doping effect and the formation of a diffusion barrier layer lack general appli-
cability. The most probable mechanism is the third, where it is proposed that the
dispersed active element oxide particles at the alloy surface act as heterogeneous
nucleation sites for the first formed oxides, thereby decreasing the internuclei
spacings. As a consequence, less time will be required for subsequent lateral
growth processes to link the nuclei to form a protective Cr
2
O
3
scale, as illustrated
schematically in Fig. 6.24.
Subsequent analytical electron microscopic (AEM), energy dispersive X-ray
analysis (EDX), and microdiffraction studies [43], in establishing the composi-
tion and structure of YCrO
3
particles formed by Y
2
O
3
-Cr
2
O
3
solid solution reac-
tion, revealed that the Y
2
O
3
and YCrO
3
particles acted as the sources of Y
3
ions
that segregated along the oxide grain boundaries in the scale formed on Ni-20%
Cr-0.6% Y
2
O
3
alloy, Y-implanted Fe-20% Cr-25% Ni stainless steel and Y-im-
planted chromium. Such observations prompted researchers to conclude that inhi-
bition of transport along the dominant grain boundary pathways by reactive ele-
ment segregants limits the oxide growth by cation diffusion. The reactive element
oxide particles act as sources for the segregants in the scale. As an extension of
this model, Pint [51] suggested the ''dynamic segregation theory,'' which states
that all of the reactive element effects in Cr
2
O
3
and Al
2
O
3
scale-forming alloy
Figure 6.24
Schematic illustration of the role of reactive element oxide particles in
an alloy surface acting as heterogeneous sites for Cr
2
O
3
nucleation and facilitating lateral
growth processes to form a protective layer.
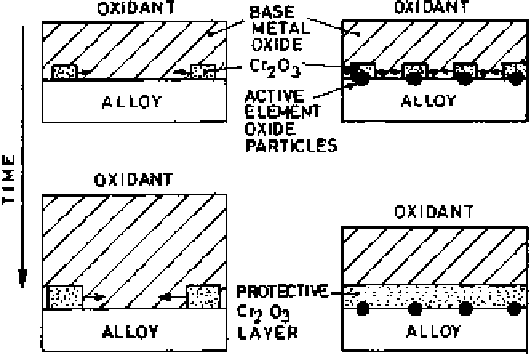