Biology Reference
In-Depth Information
using algorithms such as SHAKE [19] and RATTLE [20]. The figure
also shows that many biophysical phenomena of interest, such as pro-
tein folding, happen on time scales in the microsecond (10
−6
seconds)
to millisecond (10
−3
seconds) range. This means that the simulations
must be run for 10
9
to 10
12
time steps to simulate a single folding event.
One of the most ambitious simulations performed to date, by Duan
and Kollman [21] in 1998, was the study of the folding of a small peptide
for a microsecond.
Long time scales cause a challenge for two phases of simulations:
equilibration and sampling. First, simulations must be performed for a
long enough period of time for the material to adopt conformations
that are typical at the temperatures of interest. This is known as the
equilibration phase of a simulation, and the length of time needed for
equilibration depends on the thermodynamic conditions such as
temperature and density, as well as on the nature of the material under
study and the degree to which the starting conformation is different
from those observed in an equilibrated system. Imagine, for example,
that you begin a simulation on a peptide in its folded structure, but
at a temperature where the folded structure is unstable. It will take
some amount of time for the folded structure to unfold and adopt
conformations that are more representative of those at the temperature
of interest. Generally, the slower the time scales are in a system, the
longer it will take to equilibrate.
The second challenge caused by long time scales is that they imply
the need for longer simulations for the molecular system to adopt all
the conformations of relevance at the temperature of interest. Suppose,
for example, a molecule is known to adopt some conformation A
80% of the time and conformation B 20% of the time. We do not know
these percentages a priori, but try to determine them from running a
long simulation and looking to see what conformations the molecule
adopts. The precision with which we can determine the ratio of occur-
rences of A to those of B is determined by the number of observations
of changes of state during the simulation. If the interconversion rate
is fast, we do not need to run very long to get precise measures of
the ratio. However, if the interconversion rate is slow, we will need to
run much longer to obtain a given level of precision. This argument
holds for most properties of the system of interest. For example, if one
wishes to determine the average of the solute-solvent interaction energy,
the temporal behavior of the solute conformational change as well as of
the solvent reorganization will determine the amount of time we need to
simulate to measure this observable to any particular degree of precision.
Long time scales can usually be related to energy surfaces with
many local minima and large barriers between them. If the barriers are
large compared to the thermal energy in the system, they will not be
crossed frequently, and all thermally accessible states will not be
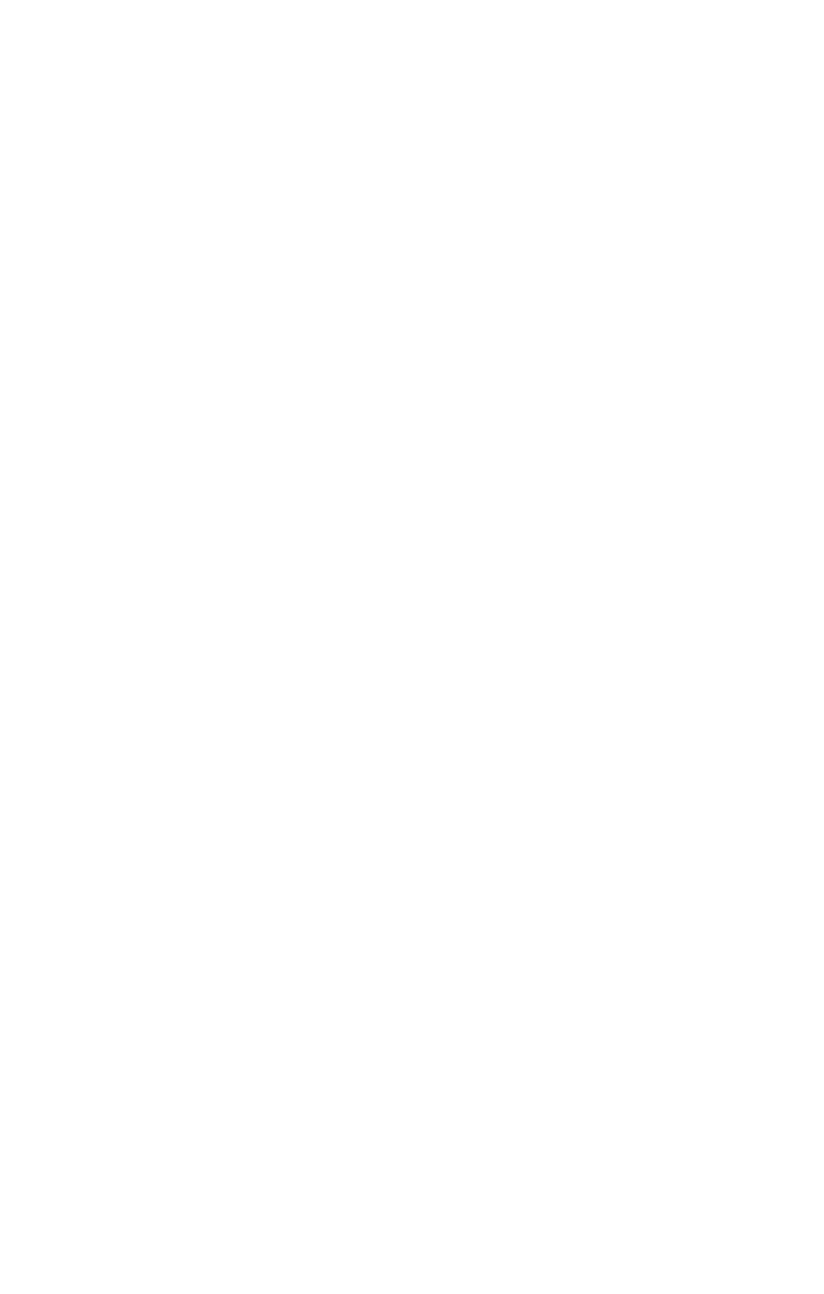
Search WWH ::

Custom Search