Environmental Engineering Reference
In-Depth Information
In this case, an explicit R can't be obtained. Because R relates the solvent (water)
velocity to the chemical (NMs) velocity, eq. 15.42 will be more useful in NM transport
calculations. However, if the adsorption/desorption process can't be assumed to be
instantaneous, then eqs. 15.42 and 15.43 can't be written; instead, eq. 15.13 should be
used. In this case, Rj in eq. 15.13 or RTR in eq. 15.38 represents the loss rate of mass due
to adsorption or deposition. If adsorption or deposition is a first-order reaction, Rj or
RTR = - kC. Otherwise, other relationships (e.g., those in Table 15.6) should be used.
Eq. 15.13 is often used to simulate experimental effluent breakthrough curves
and deposition profiles. For example, Li et al. (2008) used RTR = - k\|/C with
\\i
being a
blocking function related to a particle maximum retention capacity S
ma
x
to describe the
deposition process of nCeo in water-saturated porous media. They reported that S
ma
x
is a
function of the normalized mass flux [i.e., S
ma
x
= 1.92/(Mass flux
1
'
2
)], which provides a
means to relate the hydrodynamic effects to the magnitude of S
ma
x
under certain
experimental conditions. Results of S
ma
x
provide direct experimental evidence to
support the concept of "shadow zone" where the interplay among particle size, approach
velocity, and solution ionic strength controls the maximum attainable surface coverage
and the dynamics of deposition onto a spherical collector (e.g., the rate of clogging) (Ko
and Elimelech, 2000).
Partition and sorption of contaminants (including NMs) in environmental
systems is always a focused research area. Only a few studies were focused on sorption
and reactions of NMs with different soils or sediments, the interaction of nanosized
adsorbants and chemicals sorbed into them and how this interaction might influence
their respective chemistries in aquatic environments (USEPA, 2007). Because NMs
often have reactivity and catalytic activity to NM-mediated chemicals and often have a
tendency to form aggregates, it is possible for NMs to be retained by soil mainly via
adsorption, absorption, and transformation (e.g., precipitation, aggregation). For
example, NMs may undergo surface reactions with Al and Fe compounds under acid
conditions. In neutral or calcareous soils, NMs would be adsorbed to surfaces of calcium
carbonate (CaCOs) and clay minerals and/or precipitate as secondary calcium-NM
minerals. Like phosphorus, the reaction of some NMs in soil could be characterized by a
fast reversible process (Freeman and Rowell, 1981; Overman and Scholtz, 1999; Hu et
al., 2006) and a slow, almost irreversible process that is mostly viewed as diffusion or
nucleation controlled precipitation (or aggregation) reaction (Freeman and Rowell,
1981; Christoffersen et al., 1989; Schoumans and Groenendijk, 2000). The adsorbed
NMs might be in an amorphous form of metal-NM and would be slowly transformed
into crystalline metal-NM or aggregates, but the slow process may also cause a
hysteresis effect of NMs sorption and desorption.
The two-step adsorption and transformation process can be modeled with
Langmuir-Hinshelwood (L-H) Model. Assuming that the reaction from A to F (Table
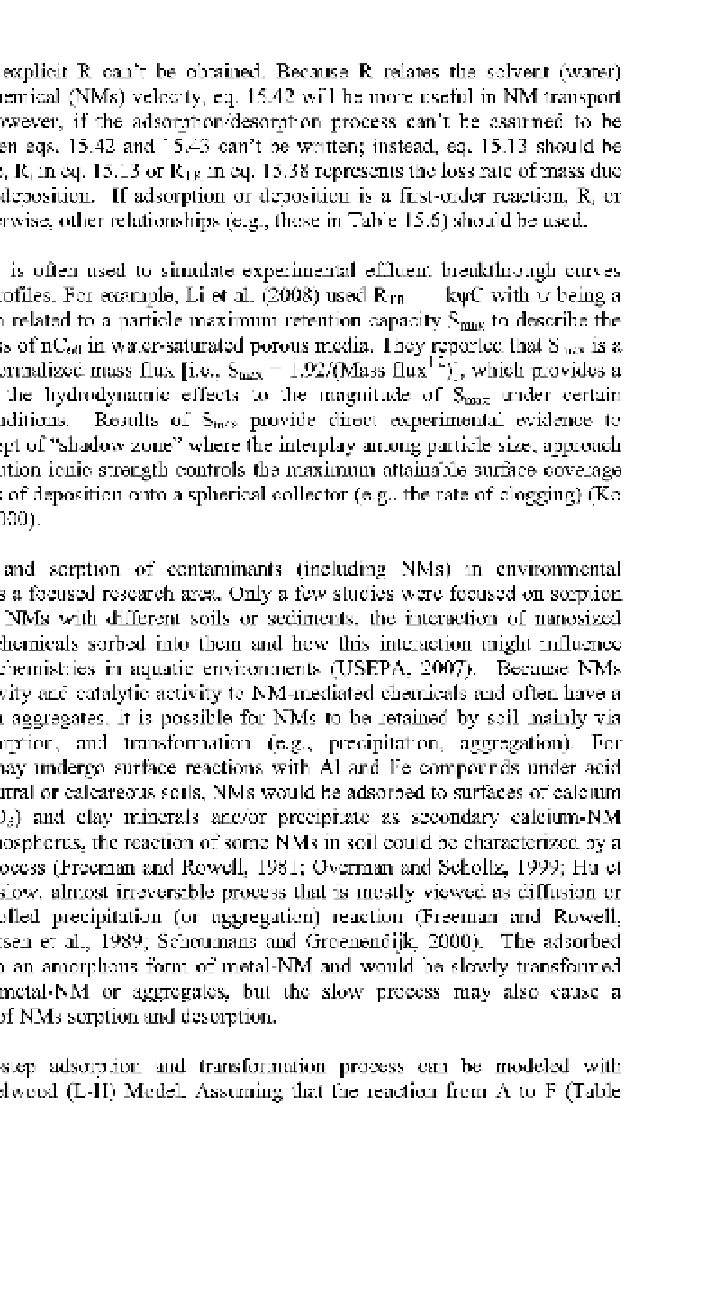
Search WWH ::

Custom Search