Geoscience Reference
In-Depth Information
Electrode Ag/AgCl
Sandbox
1
2 cm
CM
32
28
Video frame
21
14
7
Figure 5.38
Sketch of the experiment for the
localization of the Haines jumps. The sandbox is filled
with sand saturated by tap water. CM corresponds to
the common-mode range control node (reference) of
the nonpolarizing (NP) electrodes. The NP electrodes
are Ag
32 NP electrodes
Drainage tank
Valve
Saturated
sand
Capillary
fringe
AgCl electrodes with amplifiers. All the
electrical potentials are recorded relative to the
potential of the reference (CM) electrode.
-
the flow of the streaming current through a porous
medium that has a finite resistivity.
Here, we observe, for the first time, the streaming
potentials associated with Haines jumps and briefly
compare the electrical burst trends we observed with
acoustic burst trends for the same basic process,
previously studied by DiCarlo et al. (2003). We used a test
apparatus that was initially developed for EEG and was
used by Crespy et al. (2008) to observe, in real time,
the spatial distribution of very small voltages over the
surface of a saturated sandbox during very small fluid
pumping and infiltration tests. In this section, we apply
this measurement approach to the detection of the
temporally dynamic electrical bursts potentially associ-
ated with Haines jumps during drainage and imbibition
experiments.
reference (CM in Figure 5.38). All voltages measured
by this system are relative to the CM electrode. The entire
system, including the computer, was operated on bat-
teries to minimize conductive coupling with the electrical
power system (see Crespy et al., 2008, for further
explanations). It should be noted that this instrument
has also been used in numerous other investigations,
including Crespy et al. (2008), Ikard et al. (2012), and
recently Haas et al. (2013) for laboratory experiments.
After turn on, the amplified electrodes and data
acquisition system were allowed to stabilize thermally
for about 15 min. This minimized the thermal drift in
the signal due to electronics temperature stabilization.
Overall, the experiment was accomplished relatively
quickly, typically less than 5 minutes, and the thermal
mass of the sand and water was very large and
responds very slowly relative to any room temperature
fluctuations. Therefore, thermal effects on the data were
minimal.
The drainage was very simply driven by gravity
through a head difference and was started by turning
on the control valve, allowing water to flow out of the
main tank into the drainage tank (the opposite for
imbibition). The drainage tube (0.6 cm vinyl tube) was
connected near the bottom of the main tank and near
the bottom of the drainage tank (Figure 5.38), generating
a head difference between the saturated surface of the
sand and the water level in the drainage tank of 30.5 cm.
Water was imbibed into or drained from the sand through
a porous body at the bottom of the sandbox.
MATLAB code was written to read the data file gener-
ated by the data acquisition system (a BDF file format),
perform digital signal processing (DSP, using various
functions from the MATLAB Signal Processing Toolbox
5.4.2 Material and methods
The test system consists of a 20 gallon (0.075 m
3
) sand-
box filled with an unconsolidated sand (mean diameter
in the range 425
m) filled to saturation with tap
water (electrical resistivity of 650 ohm m at 25 C, mea-
surements made at 22 C). The data acquisition system
is composed of 32 nonpolarizing silver
-
600
μ
silver chloride
electrodes placed on a 5.1 cm by 5.1 cm grid forming a
5 × 7 network of electrodes at the top surface of the
sand-filled aquarium (Figure 5.38). The data acquisition
systemused in this experiment is the same systemused in
Section 5.3 (Hydraulic Fracturing Laboratory Experi-
ment), subsection 5.3.2 (Material and Method), and will
not be described again here.
The voltage reference for the measurements was con-
tained within the measurement area and was designed
into the measurement system as a DLR and voltage
-




























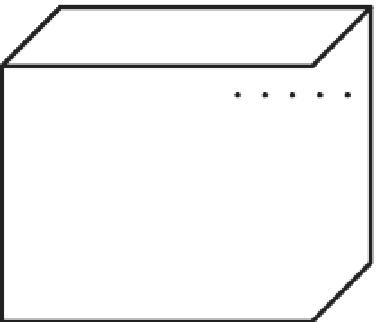




































