Geoscience Reference
In-Depth Information
are needed to check the predictive capabilities of the two
models. These new relationships also mean that the
measurement of the second Archie
10
8
Model
Data
s exponent can be
used to predict the capillary pressure curve. The hysteretic
behavior seen in the capillary pressure curve implies that a
hysteretic behavior should be present in the value of
n
.
We now turn our attention to the capillary entry pres-
sure, which can be related to the length scale,
'
10
7
Λ
,by
10
6
p
e
=
2
γ
r
c
3 210
10
5
where
represents the surface tension between water
and air (71.99 ± 0.05) × 10
−
3
Nm
−
1
and
r
c
represents
the smallest pore out of the set of pores involved in the
percolation of water through the porous material (Katz
& Thompson, 1987). Also, Katz and Thompson (1987)
developed a relationship between the permeability and
the percolation length scale,
r
c
, using percolation princi-
ples:
k
S
=
r
c
2
γ
10
4
0.001
0.01
0.1
1
10
k
S
ϕ
Figure 3.9
Comparison between the model proposed in the
main text for the capillary entry pressure, assuming that
the formation factor is related to the porosity by
F
=
2
226
F
. A comparison with
k
S
=
Λ
8
F
ϕ
−
2
yields
r
c
≈
. Using the relationship between the
length scale,
r
c
, and the permeability at saturation, we
obtain
5.3
Λ
(classical Archie
s law, Archie, 1942). The experimental data
are from Huet et al. (2005). They correspond to 89 sets of
mercury injection (Hg
'
air) capillary pressure data. Core
samples include both carbonate and sandstone lithologies.
The permeability is expressed in mD.
-
γ
226
F
k
−
0 5
2
p
e
=
3 211
S
a fully water-saturated material (Revil & Mahardika,
2013, and see Chapter 1) as
This equation can be compared to the empirical equa-
tion derived by Thomas et al. (1968),
p
e
=52
k
−
0 43
S
, with
p
e
expressed in kPa and
k
S
in mD. Archie
s law can be
used to compute
F
. Taking
m
=2.0 (a default value for
soils), and a porosity of
'
0
∂
ψ
=
Q
V
k
0
η
w
σ
C
0
= lim
ω
3 212
∂
p
j
S
=0,
=0.20 (a reasonable average
value), we obtain
p
e
=
ak
−
0 5
S
ϕ
with
a
=61 where
p
e
is
At high salinity, the conductivity can be approxi-
mated by
expressed in kPa and
k
S
in mD. We also obtain
p
e
=
b
k
S
. The proportionality between the capillary
entry pressure and the ratio,
ϕ
k
S
, is checked in
Figure 3.9. Therefore, Equation (3.199) is able to repre-
sent data but accounts explicitly for the porosity depend-
ence, which is not the case of the empirical equation
developed by Thomas et al. (1968). Note that in mercury
porosimetry, mercury is the nonwetting phase, while
water is the wetting phase.
ϕ
1
F
s
w
σ
w
σ
≈
3 213
We can now evaluate the effect of water saturation
upon the streaming potential coupling coefficient by sub-
stituting into Equation (3.212) the volumetric charge
density, the permeability, and the electrical conductivity
with their expressions that include saturation. We obtain
3.5.3 Relative coupling coefficient with the
Brooks and Corey model
The last coefficient we want to test is the streaming
potential coupling coefficient, which can be defined for
C
0
=
C
r
C
S
3 214
where
C
r
denotes the relative coupling coefficient
(a concept first introduced by Revil & Cerepi, 2004)


































































































































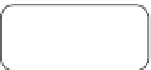











