Biology Reference
In-Depth Information
reduce GVHD caused by allogeneic T cells by perforin- and FasL-mediated
induction of apoptosis and reduction of T-cell proliferation. Fortunately, the
presence of NK cells did not interfere with the ability of the conventional T
cells to eradicate the
luc
+
A20 B cell lymphoma
[81]
.
Using a variety of luciferase positive tumors, Zakrzewski et al. evaluated the
efficacy and safety of using allogeneic T-cell precursors as immunotherapy
[82]
. The T-cell precursors were generated from bone marrow-derived hema-
topoietic stem (HS) cells by culturing them with Notch ligand-expressing bone
marrow stromal cells, Flt3-ligand and IL-7. To create mixed chimeras, BALB/c
recipients received myeloablative irradiation, syngeneic HS cells and C57BL/6
(allogeneic) T-cell precursors. This resulted in engraftment of the T-cell pre-
cursors to the thymus of recipient mice, which supported the reconstitu-
tion of primarily CD8
+
T cells as well as NK cells. Furthermore, the recipients
did not exhibit clinical or subclinical signs of GVHD. The T-cell precursors
resulted in significant anti-tumor activity as assessed via bioluminescence
imaging of
luc
+
Renca (renal cell carcinoma) and
luc
+
A20 (B cell lymphoma)
cells. GVL activity could be enhanced further by using an A20 line transduced
to express human CD19 and T-cell precursors engineered to express a chime-
ric antigen receptor targeted towards human CD19. This study has clinical
significance as it demonstrates a method of transferring potent anti-tumor
T-cell precursors into patients which can be cultured to great numbers from a
major MHC-mismatched donor, without causing GVHD.
68
In vivo fluorescence imaging
Fluorescence imaging is based on the absorption of light of one wavelength
by a fluorophore, followed by re-emission at a different wavelength.
In vivo
imaging can be performed by the use of fluorescent dye labeling of cells,
or by using cells which express a fluorescent protein. The most commonly
used fluorescent protein is enhanced green fluorescent protein (eGFP)
which has a maximal excitation of 488 nm and an emission at 509 nm. The
addition of new fluorescent proteins with spectra from 447 nm to 657 nm
has allowed simultaneous imaging of up to three or four colors in a single
cell
[83]
. Another significant advancement in fluorescence technology is the
development of quantum dots which have superior optical properties when
compared to organic dyes. Quantum dots have improved quantum yields,
higher extinction coefficients, and are more resistant to degradation and
photobleaching.
Fluorescence imaging is often limited by the optical properties of tissues and
absorption by biologicals such as hemoglobin. If using dyes or fluorescent
proteins which emit in the blue to green range, signals can only be detected
from a depth of a few millimeters. Additionally, many tissues are autofluo-
rescent, particularly in the visible range (<600 nm), which can increase the
difficulty of detecting signal above noise. Red-shifted wavelengths have bet-
ter tissue penetrating ability; however, water and lipids, which are transpar-
ent to visible light, will absorb in the infrared range. Together, these factors
make the near infra red optical window (650-900 nm) the optimal choice for
in vivo
imaging. Recently, several new probes have been developed in this
range which can be used for deep tissue imaging in small animals
[84,85]
.
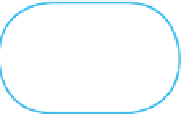
Search WWH ::

Custom Search