Environmental Engineering Reference
In-Depth Information
front. The predicted rate of spread was between 6 and
32 km/year, and the observed spread ranged between 1
and 25 km/year. Thus, at least in this case, the reaction-
diffusionmodel offers clear and testable hypotheses about
the movement of organisms.
Studies of the spread of invasive species have, however,
challenged the predictions of reaction-diffusion models.
It is increasingly obvious that rare, long-distance dis-
persal, coupled with spatio-temporal heterogeneity in
the environment, are central to the spread process, yet
reaction-diffusion models struggle to incorporate these
(Hastings
et al
., 2005b). A well known example of the
inability of classical reaction-diffusion models to pre-
dict spread (albeit for plants) is 'Reid's paradox' - the
Holocene range shifts of some forest tree species in
the northern hemisphere seems to be much quicker
than classical models predict. Accurate prediction of
such expansions requires explicit consideration of long-
distance dispersal and environmental variability (Clark
et al
., 1998).
increasingly relevant to natural landscapes, which are
becoming highly fragmented, forcing once contiguous
populations into a metapopulation structure.
Levins (1969) described the first metapopulation
model. Levins' model considers an implicit landscape in
which sites may either be occupied or empty. The model
may be either as a metapopulation model with each site
representing the occurrence of individual populations
that may become locally extinct (Hanski, 1991), or,
alternatively, each site may be viewed as the occurrence
of individual organisms in a single population with each
site being the size required by one adult individual of the
species of interest (Tilman
et al
., 1994, 1997). Either way,
the model is formulated as:
dp
dt
=
−
−
cp
(1
p
)
mp
(13.3)
The change in site occupancy over time (
dp/dt
)isa
function of the rate of per-site colonist production (
c
),
the proportion of empty or available sites (1
−
p
), and
the per-site mortality rate (
m
). The metapopulation will
persist if
c
>
m
, and when
c
>
m
,
p
approaches a globally
2. Metapopulation and patch-occupancy models
The use of the 'metapopulation' as a framework for
spatial population modelling has become popular since
the mid-1990s (Hanski, 1998). A metapopulation refers
to a group of spatially separated local populations that
exchange individuals infrequently (every two to three
generations). Despite the repeated extinction of local
populations, a metapopulation may persist indefinitely
so long as empty patches are recolonized at a rate greater
than that at which occupied patches suffer extinction
(Figure 13.1). This conceptualization of populations is
stable equilibrium (
p
*) at 1
c
. (Figure 13.2).
Tilman
et al
. (1994) extended the basicmetapopulation
model to include habitat loss:
dp
dt
=
−
m
/
cp
(1
−
h
−
p
)
−
mp
(13.4)
where:
h
is the proportion of habitat in the landscape lost
and so not available for colonization.
In this modified form of the model, the globally sta-
ble occupancy equilibrium (
p
*) is 1-
m
/
c
-
h
.Thus,the
metapopulation will persist so long as
c
m
and the
amount of habitat loss (
h
) does not exceed 1 -
m
>
/
c
Time = 1
Time = 2
Time = 3
Time = 4
Figure 13.1
Schematic view of the metapopulation concept; circles represent patches of habitat of different size and isolation. Patches
periodically suffer extinction and become empty (grey) and are periodically recolonized and occupied (black); patches with dotted
outlines have suffered extinction since the last time-step; arrows indicate directions of patch recolonization. The theory of island
biogeography predicts that smaller and more isolated patches will suffer higher rates of extinction and lower rates of recolonization
than larger and less isolated ones. Although no single patch is constantly occupied so long as the rate of colonisation exceeds that of
extinction the metapopulation (i.e. the sum of the local populations) remains intact.
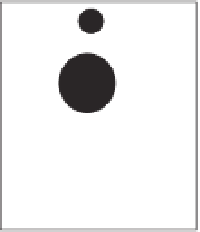







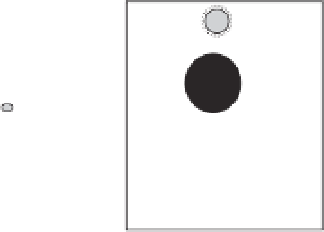
















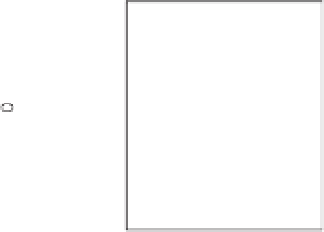
















Search WWH ::

Custom Search