Biology Reference
In-Depth Information
The first engineering for taxadiene production in
E. coli
employed overexpression of IPP
isomerase, GGPPS, TS, and DXP synthase, the first enzyme of the DXP pathway. An initial
titer of 1.3 mg/L taxadiene was formed.
187
Extensive pathway engineering was carried out
by Ajikumar and coworkers in which the taxadiene pathway was separated into an
upstream module, consisting of four of the eight DXP pathway enzymes that were
reported to be rate-limiting, and a downstream module, consisting of GGPPS and TS. The
expression of each module was varied by different promoters and plasmid copy numbers.
Thirty-two total combinations of the modulated upstream and downstream modules
were tested for taxadiene production, the maximum producing nearly 300 mg/L by shake-
flask and over 1 g/L by fed-batch reactor.
188
Taxadiene-5
α
-ol hydroxylase (T5
α
OH), the
P450 that oxidizes taxadiene to taxadiene-5
α
-ol, was engineered with its partner
reductase (TCPR). Optimal taxadiene-5
-ol production (24 mg/L in shake-flask, 58 mg/L
in bioreactor) was found with an
N
-terminal engineered T5
α
α
OH linked to a 74-amino-
acid truncated TCPR.
CONCLUSIONS AND FUTURE PERSPECTIVES
The potential applications of synthetic biology to the pharmaceutical industry are
far-reaching, from the earliest stages of compound discovery through lead optimization
and industrial-scale production. As we have demonstrated in this chapter, a vast array of
computational tools is now available for tasks ranging from gene and pathway discovery
all the way to production host optimization. Additionally, sophisticated and facile
experimental tools have been developed that make the manipulation of biosynthetic
pathways and even entire genomes routine. Application of these tools and synthetic biology
concepts has already led to the discovery of new and interesting natural products from
diverse environments. Further, engineering and combinatorial approaches enable the
biosynthesis of
natural products not found in nature. We have already seen the
application of synthetic biology techniques make significant contributions to the production
of valuable drugs such as erythromycin A, artemisinin, and taxol; and countless similar
examples are likely to appear in the coming years. As a result, synthetic biology is poised
to be a major driving force in the pharmaceutical industry, unlocking as-yet undiscovered
drug molecules and transforming the perception of the cell from an ill-defined black box to
a programmable, tunable machine.
'
unnatural
'
199
Acknowledgments
We thank the National Institutes of Health (GM077596), the National Academies Keck Futures Initiative on
Synthetic Biology, the Energy Biosciences Institute, and the National Science Foundation as part of the Center for
Enabling New Technologies through Catalysis (CENTC), CHE-0650456 for financial support in our synthetic
biology projects. Y. Luo also acknowledges fellowship support from the National Research Foundation of Korea
(NRFK) (220-2009-1-D00033).
References
1. Newman DJ, Cragg GM. Natural products as sources of new drugs over the last 25 years.
J Nat Prod
.
2007;70:461
477.
2. Fickett JW. Recognition of protein coding regions in DNA sequences.
Nucleic Acids Res
. 1982;10:5303
5318.
3. Borodovsky M, McIninch J. GENMARK: parallel gene recognition for both DNA strands.
Compu Chem
.
1993;17:123
133.
4. Lukashin AV, Borodovsky M. GeneMark.hmm: new solutions for gene finding.
Nucleic Acids Res
.
1998;26:1107
1115.
5. Besemer J, Lomsadze A, Borodovsky M. GeneMarkS: a self-training method for prediction of gene starts in
microbial genomes. Implications for finding sequence motifs in regulatory regions.
Nucleic Acids Res
.
2001;29:2607
2618.
6. Besemer J, Borodovsky M. GeneMark: web software for gene finding in prokaryotes, eukaryotes and viruses.
Nucleic Acids Res
. 2005;33:W451
454.

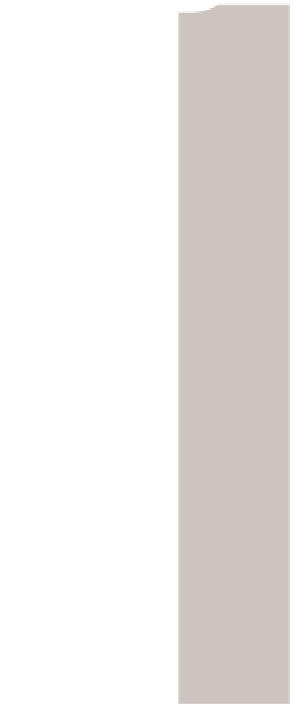