Biology Reference
In-Depth Information
transcription factor continues to activate its own expression even when the stimulus is later
absent, resulting in cellular memory.
Oscillators
In nature, cells exhibit a variety of oscillatory functions such as the cell cycle and circadian
clock. Genetic oscillators are of particular interest to synthetic biology because these can be
employed to precisely synchronize and time the expression of proteins and RNA for both
therapeutic and nontherapeutic applications. The first synthetic genetic oscillators were
reported in prokaryotic systems. The simplest example, reported by Goodwin
68
in 1963, just
comprised a single gene (LacI) that represses itself. This was followed by the construction of
more complex repressilators, which are regulatory networks of more than one gene, with
each gene repressing its successor in the cycle.
6
The first synthetic repressilator was
developed by Elowitz and Leibler.
6
This involved a closed loop of three genes consecutively
repressing each other, with LacI repressing tetR, which represses
λ
cI, which in turn represses
LacI gene, thus completing the loop.
Both the Goodwin oscillator and repressilators function through repression of gene
function. The next step in oscillator design would be to incorporate both activation and
repression of gene expression. This was realized by the construction of an amplified
negative-feedback oscillator by Atkinson et al.
54
This consisted of one gene (NRI) promoting
its own transcription via a positive self-feedback loop, which also promoted the
transcription of a second gene (LacI), which in turn repressed expression of the first gene
(NRI), forming a negative feedback loop. Subsequently, more complex synthetic gene
oscillators with tunable function,
69
and which utilizes metabolic flux as a control factor in
system-wide oscillation,
70
were constructed in
E. coli
.
The first synthetic genetic oscillator to be constructed in mammalian systems was reported
by Tigges et al.
71
This is, in fact, an amplified negative-feedback oscillator that contains a
delay in the negative-feedback loop. The resultant effect is that the oscillations in gene
expression are undamped. This system is composed of two genes, with both sense and
antisense transcription occurring from one of the genes. The sense transcript is translated
into a protein (tTA) that promotes its own transcription and that of a second gene (PIT),
which in turns activates antisense transcription from the first gene (tTA). This antisense
transcript will then repress translation of the sense transcript through hybridization,
constituting a negative feedback loop. Following up on this study, Tigges and colleagues
constructed a low-frequency variant of this oscillator.
72
To date, these are the only synthetic
genetic oscillators to be reported in mammalian systems.
163
Filters
In synthetic biology, filters and bandpasses are required for noise control, as well as for
controlling the output of specific signal modes. The study of Austin et al.
73
with
E. coli
cultures demonstrated that negative feedback is able to shape noise spectrum. In the study
of Hooshangi et al.,
74
low-pass filtering of input signals was achieved in a synthetic
transcriptional cascade within bacterial cells. This conferred some robustness to fluctuations
in input signal conditions, that is, noise. The first synthetic bandpass filter in bacterial
systems was originally reported by Basu et al.,
75
in which one cell population (receiver) was
programmed to respond to a defined concentration range of acyl-homoserine lactone (AHL)
produced by another cell population (sender). Subsequently, Sohka et al.
76
developed a
bacterial bandpass filter with tunable function. Tunability of the bandpass filter was
achieved by utilizing three different
-lactamase genes that conferred different levels of
ampicillin resistance.
76
A more complex bacterial bandpass filter incorporating metabolite-
responsive riboswitches was reported by Muranaka and Yokobayashi.
77
This comprised of
β

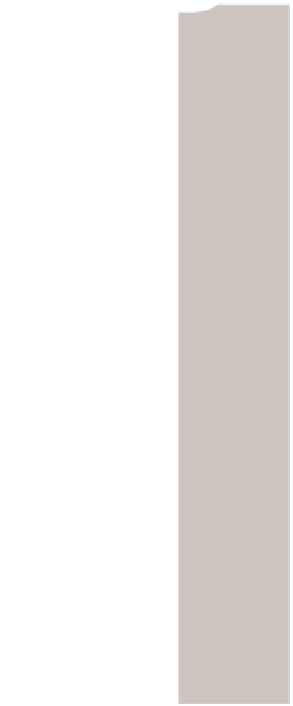