Biology Reference
In-Depth Information
iterations of sequence design and gradient-based minimization of the designed sequence are
carried out. During this stage, the original theozyme residues are not allowed to change
their identities anymore, and the energy function is augmented with terms that ensure that
the theozyme residues stay in their desired theozyme geometry.
35
If stochastic side-chain
placement algorithms are used, this stage is usually carried out several dozen times for each
match. Depending on the initial number of matches, several 100
10 000 design models are
generated. The models are then usually filtered and ranked according to several criteria
reflecting the degree of realization of the ideal theozyme geometry, the affinity of the
designed site for the new ligand, and the structural integrity of the scaffold.
The current strategy of designing a novel catalyst by placing a theozyme into a known
protein structure is rooted in two observations. First, many enzymological studies have
shown that the catalytic prowess of natural enzymes is usually caused by a small number of
catalytic residues, while many other residues that are also found in the active site play a far
less important role in catalysis.
46,47
Knocking out the catalytic residues, i.e. replacing them
with chemically inert alanine, will have a huge effect on efficiency of the enzyme, while
changing other binding site residues to Ala will often have no influence on the activity at
all. One conclusion from this observation is that to design an active site, it is most
important to properly position a handful of key catalytic residues. Second, computational
protein design as a technique is still far from perfect, and, despite Kuhlman et al.
s de novo
fold design work,
48
it is still unclear whether current algorithms and force fields are reliably
capable of designing sequences that fold into a given protein architecture or fold. Further, as
of yet there are no algorithms that would design a reasonable protein backbone
conformation starting from a theozyme only. Placing a theozyme into an already known
structure and then designing only the surrounding site, which usually means mutating less
than 10% of the scaffold residues, circumvents both of these problems. Since the backbone
structure is given by the scaffold there is no need to come up with a new one, and since the
final designed sequence is highly homologous to the wild-type scaffold sequence, one can
reasonably assume that the designed protein will be expressed and folded.
'
115
Several examples of successful de novo computational enzyme design have been
accomplished in recent years. These include two reports
49,50
of designed catalysts for the
Kemp elimination, a well-studied model reaction, one set of proteins catalyzing a retro-
aldol reaction through a similar mechanism as natural aldolases,
51
and one study reporting
the design of a
52
catalyzing the Diels-Alder reaction, which is a
Diels-alderase
'
'
carbon
carbon bond forming reaction for which no natural enzymes exist. The Kemp
elimination is an isoxazole ring opening reaction that can be initiated by deprotonation of
the carbon adjacent to the azole nitrogen, and the substrate used in both studies featured a
benzyl ring adjacent to the isoxazole moiety. In the transition state, negative charge
accumulates on the isoxazole oxygen. Thus, for the Kemp eliminases, in both studies the
theozyme consisted of three elements: a base (either a Glu/Asp or His) to deprotonate the
relevant carbon; a hydrogen-bond donor to stabilize the isoxazole oxygen; and an aromatic
side-chain (Phe/Tyr/Trp) to stack against the substrate
s phenyl group and thus aid with
binding the substrate. In the work by Roethlisberer et al.,
49
a scaffold library was then
scanned for matches to this theozyme, and after designing the sequence and ranking the
resulting matches, 59 designs were selected for expression. Eight of these designs showed
detectable activity, and for each of the designs, replacing the catalytic base by a nonprotic
residue resulted in a significant decrease of the catalytic activity. In the second study by
Privett et al.
50
a slightly different approach was followed. Instead of searching through a
number of scaffolds, the authors identified an aspartate in a hydrophobic cleft of a xylanase,
and chose this residue to be the catalytic base. The substrate was placed in a position to
interact with this residue, and the surrounding pocket was then designed to accommodate
the substrate in this position. The resulting design had activity comparable to the designs
reported by Roethlisberger et al.
'

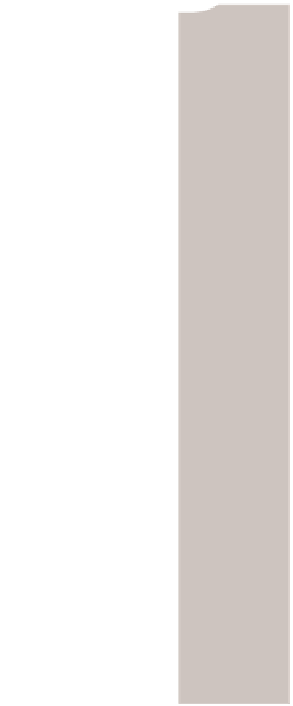