Biomedical Engineering Reference
In-Depth Information
1
0.8
179 ΜM
0.6
C
BIL,OUTLET
C
BIL,INLET
140 ΜM
0.4
90 ΜM
C
alb
0.2
0
0
50
100
150
200
250
300
350
400
450
T [MIN]
Figure 5. Typical bilirubin breakthrough curves on anionic resin.
C
ALB
: albumin concentra-
tion in the solution; lines: model fitting; circles: experimental data. Operating conditions:
feed flow-rate 1 ml/min ; resin mass 3 g ; inlet bilirubin concentration
C
BIL,INLET
=100 µM.
where
N
∗
BIL
is the solute adsorbed amount at equilibrium with the liquid phase, given by
equation (30),
K
C
is the LDF mass transfer coefficient and
R
is the radius of adsorbent
particles.
Equations (34) and (35) can be integrated with the following initial and boundary con-
ditions:
T = 0
0≤Z≤H
C
BIL
= 0 ;
N
BIL
= 0
(36)
V C
I
BIL
=−D
Z
∂C
BIL
T > 0,
Z = 0,
+ V C
BIL
(37)
∂Z
∂C
BIL
∂Z
T > 0
Z = 1
= 0
(38)
where
C
I
BIL
is the bilirubin concentration in the inlet solution.
The system of equations (34) to (38) allows to predict bilirubin breakthrough curves,
once the axial dispersion coefficient, equilibrium conditions and mass transfer coefficient
are known. As for the dispersion coefficient
D
Z
, the correlation proposed by Chung and
Wen (1968) was used. The LDF mass transfer coefficient
K
C
is a lumped parameter that,
from a general point of view, accounts for the diffusional resistance offered by adsorbent
particles and the convective resistance in the liquid film surrounding the particles. Due to
the intrinsic semi-empirical nature of the LDF model,
K
C
must be regarded as an adjustable
parameter that can be obtained by fitting of experimental data. In this case, in order to
improve the model adaptability to the experimental data, a modified form of LDF kinetics
was considered, assuming
K
C
to be a decreasing function of time
K
C
1 + AT
B
K
C
(T) =
(39)



































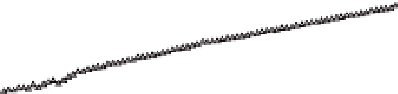
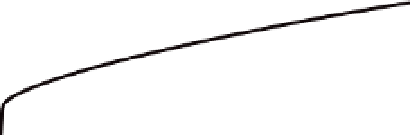

















































































































































































































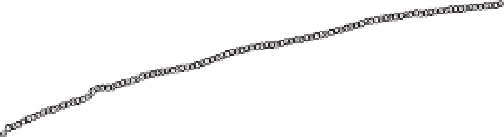
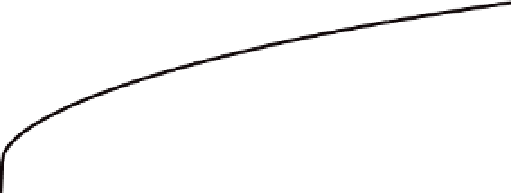



