Environmental Engineering Reference
In-Depth Information
The NO emissions decreased with the decrease of equivalence ratio as a direct
result of lower combustion temperature for all the cases presented here. Note that
the trends for CO agree favorably well with the previously reported results
(Lefebvre
1995
). Emission of CO diminishes with increase in equivalence ratio,
reaching a minimum value. Beyond this point, any further increase in the equiva-
lence ratio causes CO emissions to increase. The observed high levels of CO at low
equivalence ratios are due to the slow rates of oxidation associated with low
combustion temperatures. However,
guration CA, the observed CO
emission at equivalence ratio of 0.5 is lower than the observed emissions for the
other con
for con
gurations examined. Such behavior indicates that the reaction rate is
higher at higher temperatures. This suggests that the combustion occurs in a less
diluted oxidizer (higher oxygen concentration) so that colorless distributed condi-
tions are not satis
ed. NO emission for this con
guration, compared to other
con
gurations, was found to be higher to reveal the effect of higher reaction rate
and temperature encountered in this con
guration.
An increase in the equivalence ratio raised the
flame temperature, which
accelerated the rate of oxidation to subsequently decrease the CO emissions.
However, at temperatures higher than around 1,800 K, the production of CO by
chemical dissociation of CO
2
starts to become signi
fl
cant. Thus, at high equivalence
ratios, CO levels are high because they attain equilibrium values.
The radical intensity distribution of OH* chemiluminescence showed that the
reaction zone is in the shape of a crescent. It can be seen that as the fuel introduction
method is changed, the reaction zone moves gradually downstream. Premixed
combustion case
resulted in the earliest reaction zone, whereas separate air
and fuel introduction as in case
“
PR
”
“
NP1
”
resulted in a longer ignition delay. OH* zone
movement, which can be seen as a
flame marker, outlines the difference between
mixing and ignition delay in each case discussed here. Figure
6
shows the OH*
chemiluminescence intensity distribution at an equivalence ratio of 0.8 for the
different cases presented here. A similar trend was also observed at other equiva-
lence ratios.
Numerical simulations were carried out to help develop an understanding of the
difference in behavior between each of the fuel injection scenarios discussed herein.
The calculated results showed that, for each fuel injection scenario, the ignition of
fl
A+F
A+F
A
A
F
F
PR, Phi=0.8
CA, Phi=0.8
NP0, Phi=0.8
NP1, Phi=0.8
Fig. 6 OH* chemiluminescence intensity distribution for different injection arrangements

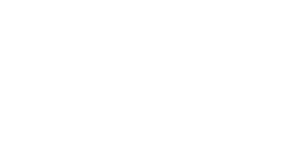














Search WWH ::

Custom Search